Metallic cations are essential in biological systems, in particular in the role they play in proteins. About half of the proteins expressed in humans interact with metals—the complete set of these biomolecules containing a metal is referred to as the metallome. Even though most metals essential to the metallome are found in the upper part of the periodic table, they can be replaced by other metals that carry out no physiological function, inducing a variety of physiological and toxicological effects. These metals devoid of physiological function include the actinides, notably plutonium. From a physics standpoint, due to the instability of all of its isotopes, plutonium presents a radiological toxicity that is totally independent of its chemical properties. Chemically, plutonium is a hard acid according to the Pearson classification and therefore it exhibits special affinity for hard bases such as hydroxides, carbonates, carboxylates, or phosphonates, and consequently for certain groups found in proteins, such as tyrosine, aspartate, or histidine.
Plutonium(IV) presents very low clearance and is very strongly retained in the human body. Biodistribution studies indicate that more than 80% of the plutonium retained is partitioned between two main organs: the skeleton and, preferentially, the liver. Human epidemiological data are very scarce—fortunately—but this paucity means that the toxicity of plutonium has had to be estimated using experiments performed on animal models. Furthermore, it remains difficult to quantify plutonium in the skeleton due to individual variability (age, health status, etc.), the small size of bone samples available for analysis and their heterogeneous origin (sternum, femur, ribs, etc.), and the time elapsed between autopsy and postmortem sampling. All of these parameters require extrapolations that contribute to the overall heterogeneity of the results.
As early as 1965, transferrin (Tf) was one of the very first target proteins identified; its modes of interaction with the actinides remain widely explored to this day. But transferrin by itself cannot explain why plutonium accumulates in the bones. Bone is composed of a network of collagenous and non-collagenous proteins permeated with a mineral matrix of hydroxyapatite. While fetuin-A (Fet) and albumin represent more than 25% of the non-collagenous proteins present in the serum, fetuin-A is four times more concentrated than albumin in the calcified matrix.
Given the special properties of these two proteins—one of them produced by the liver (transferrin) and the other ending its lifespan in the bone matrix (fetuin-A)—we considered it relevant to study their affinity for plutonium and examine whether they could enter into competition with each other.
Figure 1. Transferrin is a glycoprotein with a molecular weight of approximately 80 kDa (kilodalton). Its concentration in the blood is approximately 2.5 mg/mL (≈ 30 μM). It is responsible for transporting iron in the blood to the organs, particularly the liver. Two binding sites are found on the two lobes of this protein: the C-lobe (log10 K(FeIII) = 21.4) and the N-lobe (log10 K(FeIII) = 20.3). The carbonate anion, which acts as a synergetic anion, is necessary for cation fixation and thereby enables the closure of the lobes. Only the diferric form (referred to as holotransferrin) adopts the conformation required for internalization in the cell. The free form (without iron, known as apotransferrin) and the monoferric form, which together represent 70% of total blood protein, can bind with plutonium. The half-life of transferrin in the blood is roughly eight days.
Figure 2. Human fetuin (also known as fetuin A or α2-HS-glycoprotein) is a protein with a molecular weight of 48 kDa, but its highly glycosylated nature can increase its apparent weight to approximately 56 kDa. It consists of three domains: two cystatin domains known as D1 and D2, and an unstructured domain (D3). Fetuin is a plasma protein derived from the liver. It is abundant in fetuses and young children, decreasing over time to about 15 μM in adults. Its function is to control the formation of calcium phosphate clusters by binding them and transporting them to the bone matrix, while its life cycle comes to an end 1.5 days after its release into the bloodstream.
Figure 3. Effect of increasing (a) Fet and (b) Tf concentrations on the Pu(NTA)2 complex (NTA = nitrilotriacetic acid). The relative area of Pu(NTA)2 (black spheres) is shown as a function of the relative area of PuFet (red spheres) or of PuTf (apo form, blue spheres). By locating the point at which both species present the same concentration, i.e., [Fet] = (9.1 ± 1.3) μM and [Tf ] = (5.2 ± 1.5) μM, it becomes possible to calculate the binding constant:
log K = 26.20 ± 0.24 for fetuin and 26.44 ± 0.28 for transferrin.
Serum proteins: Transferrin and fetuin
Transferrin was discovered by Holmberg and Laurell in 1947. This glycoprotein of approximately 80 kDa in molecular weight (Fig. 1) can bind up to two Fe(III) cations in two different lobes (known as the C-lobe and the N-lobe), which leads to the closure of the lobes, the recognition of transferrin by its receptor, and the cellular internalization of the transferrin. Ongoing studies have established the interaction of this protein with actinides in oxidation states III, IV, V, and VI and identified its behavioral similarities with Fe(III). The binding sites of actinides are common to those of Fe(III), involving histidine, tyrosine, and aspartate groups.
Fetuin-A is a non-collagenous protein of approximately 48 kDa (Fig. 2), discovered by Pederson in 1944. It can bind to a great many receptors with various functions; recently, its strong affinity for uranium(VI) was demonstrated. It possesses three known binding sites for this actinide, including a site with particularly high affinity that has not yet been clearly identified, but appears to be located in the first cystatin domain (D1). Its affinity for plutonium has been unknown up to the present time.
Stability constants of transferrin and fetuin with plutonium
It is extremely difficult to study plutonium(IV) in physiological environments due to competing hydrolysis reactions. For plutonium(IV) to be stabilized at physiological pH (7.4), it must be protected by a chelate whose affinity for plutonium overcomes the issue of hydrolysis reactions. We opted for the use of nitrilotriacetic acid (NTA), which at a concentration of 2 μM makes it possible to study the binding process with transferrin and fetuin up to a pH of 7. This investigation relies on capillary electrophoresis (CE) coupled to inductively coupled plasma (ICP) mass spectrometry, a method based on the competition between the Pu(NTA)2 complex and the proteins, whose concentrations range from 0.1 μM to 0.1 mM. Examples of these variations are provided in Fig. 3, while typical electropherograms are shown in Fig. 4.
Figure 4. Electrophoregrams obtained via CE-ICPMS coupling for (a) a [Fet]/[NTA] ratio of 4 and (b) a [Tf ]/[NTA] ratio of 5. Two peaks are associated with fetuin (which presents two different conformations with the same affinity for plutonium(IV)), while only one peak is detected for transferrin (only one conformation of the apo form). It can be noted that for similar protein concentrations (8 μM for Fet, and 10 μM for Tf ) and an NTA concentration of 2μM, Tf binds to plutonium(IV) more strongly than does Fet.
Fetuin and transferrin as potential vectors for Pu accumulation in the liver and bones
The theoretical biodistribution of plutonium between the liver and the bones can be estimated using the relative affinities of transferrin and fetuin for plutonium. Based on average blood concentrations of fetuin-A (15 μM) and unsaturated transferrin (70% of 30 μM), the relative distribution of plutonium between these two proteins depends on the ratio of their binding constants (1026.44/1026.20) and on the ratio of their concentrations in the bloodstream (70% × 30 μM/15 μM). The value of this ratio is about 2.5, i.e., a relative distribution of 71% for Pu-Tf and 29% for Pu-Fet. This partition agrees with experimental observations favoring the liver, but the concentrations of these proteins can vary from one individual to another. Because transferrin concentrations in healthy subjects range from 25 to 51 μM (or from 17.5 to 35.7 μM for the unsaturated forms), the resulting Pu-Tf/Pu-Fet ratios range from approximately 2 to 4, corresponding to partitions between 67/33 and 80/20. A similar observation can be made for fetuin, whose concentration can vary between 8 and 21 μM in healthy subjects.
These results must be compared with the in vivo data available in the literature. Stather and Nenot collected data with approximately 200 samples showing that the distribution between the liver and bones was between 0.57 and 0.23 pCi.kg–1, respectively, giving a partition of 71/29—identical to the result calculated using binding constants. In addition, five studies carried out in the 1970s using 25 autopsies of highly contaminated workers revealed an average relative distribution of 72% in the liver and 28% in the bones, but with significant variation among individuals (16–95% for the liver; 5–85% for the bones).
Thus, the physiological variations of transferrin and fetuin concentrations among individuals can, to a certain degree, also help explain these heterogeneous plutonium distributions. Importantly, fetuin-A concentration in infants and young children can exceed 20 μM, possibly explaining the greater plutonium accumulation observed in the bones of young organisms.
Conclusion
Because capillary electrophoresis is a non-intrusive technique (speciation is preserved), its coupling with ICP-MS provides an ideal tool for studying plutonium-protein interactions. Studies focusing on the interactions of plutonium with transferrin and fetuin-A proteins at near-physiological pH have been successful, supplying valuable information indicating that the driving force between these two proteins is primarily thermodynamic. This observation has led us to propose that the fate of plutonium in a body depends mainly on the relative concentrations of these two proteins in the bloodstream. Fetuin-A is highly concentrated in the bone matrix and its strong affinity for plutonium could now provide a convincing explanation for plutonium accumulation in the bones. We therefore suggest that fetuin-A is one of the carriers (serum citrate is another) that transport plutonium to the bones.
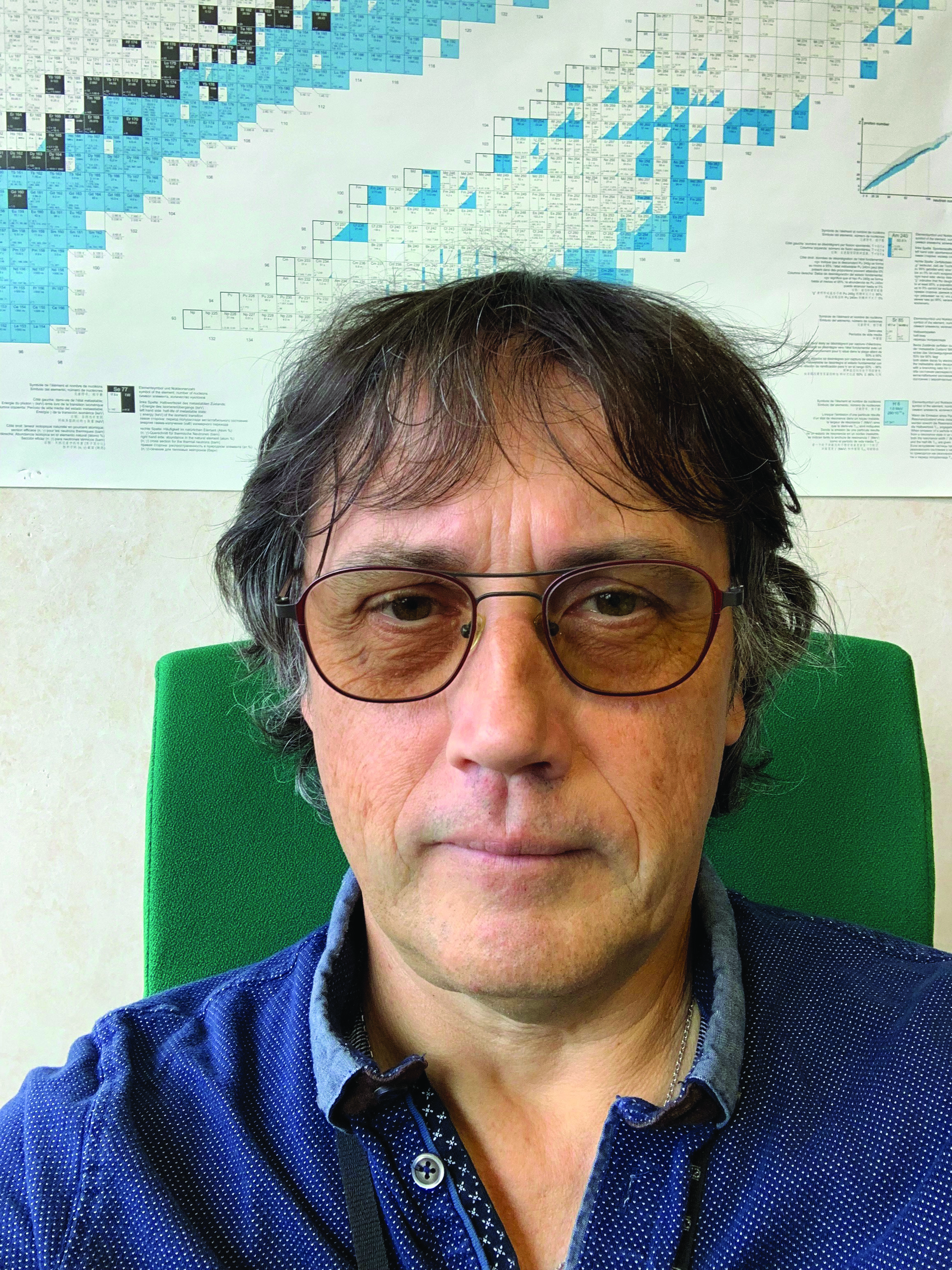
Jean Aupiais is currently Senior Scientist at CEA (the French Alternative Energies and Atomic Energy Commission) DAM Ile-de- France and Professor of Radiochemistry at the National Institute of Nuclear Science and Technology. His research interests are focused on actinide speciation by means of hyphenated techniques, thermodynamics of actinides in solution, and detection of radionuclides at the ultratrace scale.