Tuberculosis, Pneumonia, Meningitis, Flesh-Eating Disease—all potentially fatal, all commonly caused by drug-resistant bacterial infections. More Americans die from such infections—usually acquired during an unrelated hospital visit—than from traffic accidents, guns, and HIV combined. And the rates are climbing. In the absence of new antibiotics, doctors desperately need some other way to battle the bacteria.
As it turns out, there is another way. Laboratory chemist Stosh Kozimor is the lead scientist on a team investigating a completely different kind of treatment, taking a cue from a type of cancer therapy currently in development. The idea is to deliver radioactive isotopes directly to the harmful bacteria (or the tumor cells, in the case of cancer) within the body and thereby kill them with extremely localized irradiation. Unlike a targeted antibiotic drug that kills the bacteria but leaves human cells unharmed—obviously a great option when such a drug exists—this type of radiation is an indiscriminate short-range killer, and so the emission source must be quickly and carefully brought adjacent to the cells that actually need killing. (It will still damage some human cells, but at levels the body can handle.)
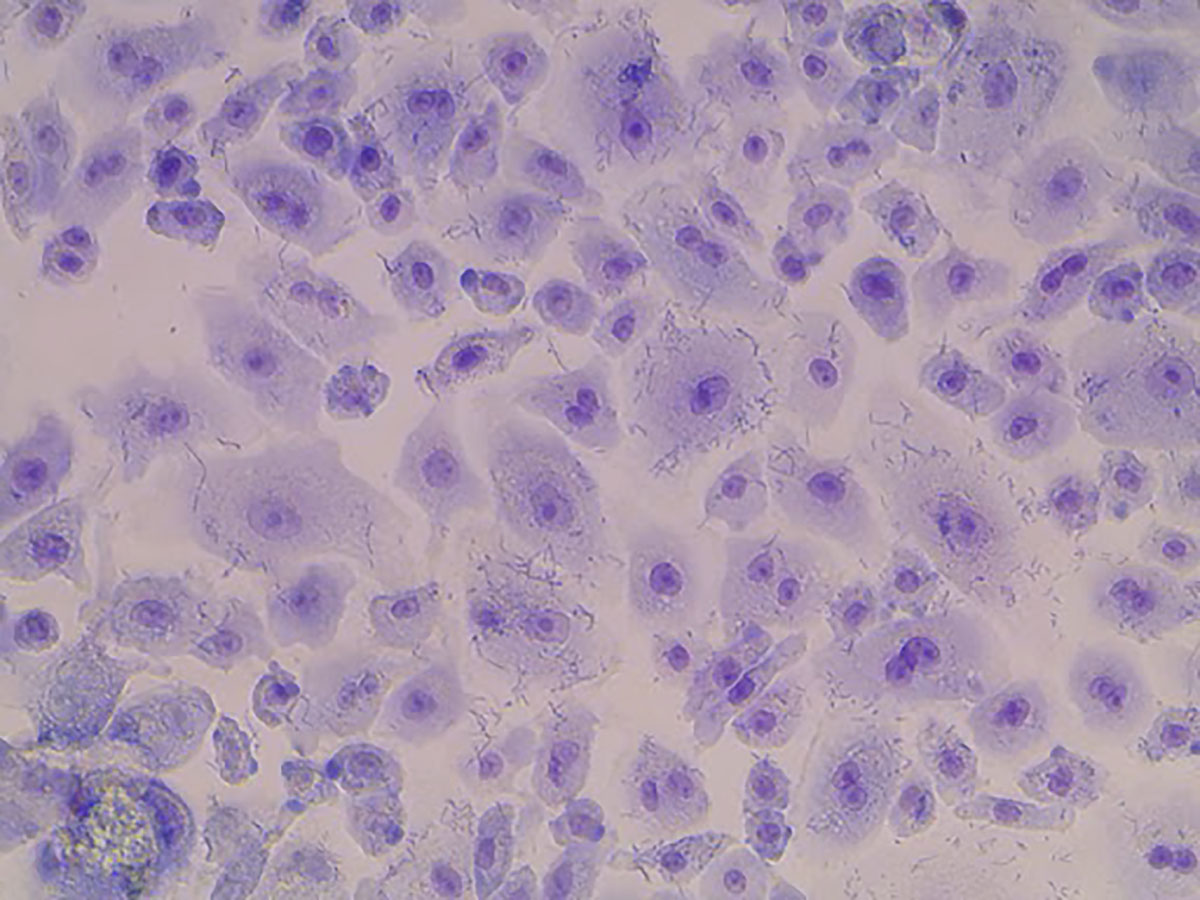
Human lung alveolar tissue infected with the vaccine strain of Y. pestis (seen here as hundreds of short, thin line segments). The bacteria infect both inside and outside alveolar cells, ultimately tearing apart the delicate connections between each cell, and can enter the bloodstream from inside the lung.
The key to making this treatment effective is threefold. “First, you need a suitable radioisotope to emit sufficient radiation—and then become inert before it does too much collateral damage,” says Kozimor. “Then you need a delivery system that will rapidly home in on the bacteria in question. Finally, you need a chemical mechanism to attach one to the other.”
The first two legs of this triad are already major Los Alamos capabilities. The Lab’s history with nuclear science and engineering has led it to develop many critical technologies, including the production of radioisotopes for medical therapies. Actinium-225, in particular, has shown tremendous promise for this type of treatment. It has a brief ten-day half-life, so it doesn’t present a long-term danger to the patient, and it decays in a powerful series of four alpha-particle emissions—a type of radiation that doesn’t penetrate far and thus is only dangerous in a very narrow radius around its target. The radioactive decay chain ultimately converts the actinium-225 into bismuth-209, which is neither radioactive nor toxic at the levels in question. Actinium-225 is not found in nature due to its short half-life and is difficult to produce, requiring a proton accelerator and a sophisticated separation process; fortunately, the Lab has both.
Alpha radiation kills only in its immediate vicinity, so the emission source must be adjacent to the cells that need killing.
The second leg, delivering the isotope to the pathogen, is another major Los Alamos success story. The delivery system is an antibody: a biomolecule that latches onto a particular complementary biomolecule, or antigen, found on the outside of the target cell, with great specificity, like lock and key. Laboratory bioscientists have developed a highly successful system for rapidly identifying specialized antibodies using a blend of computer analysis and wet-lab benchwork. One of their major antibody design efforts, for example, targets Yersinia pestis, the bacterium that causes plague, and this is the antibody Kozimor’s team used in their experiments. (Plague is treatable with existing antibiotics if caught early and not antibiotic resistant, but Y. pestis was chosen for this research to explore treatments against a germ-warfare attack. The researchers are working with its vaccine strain.)
The third and final leg is the creation of a stable chelator, or linking molecule, to join the actinium to the antibody. It must bind in a fashion that introduces no toxicity, is not simply excreted out of the patient, and is not vulnerable to having the actinium displaced by other metals found inside the body. In addition, the chelator should be designed in such a way that the methods used to create it can be easily adapted for other antibodies meant to treat other pathogens.
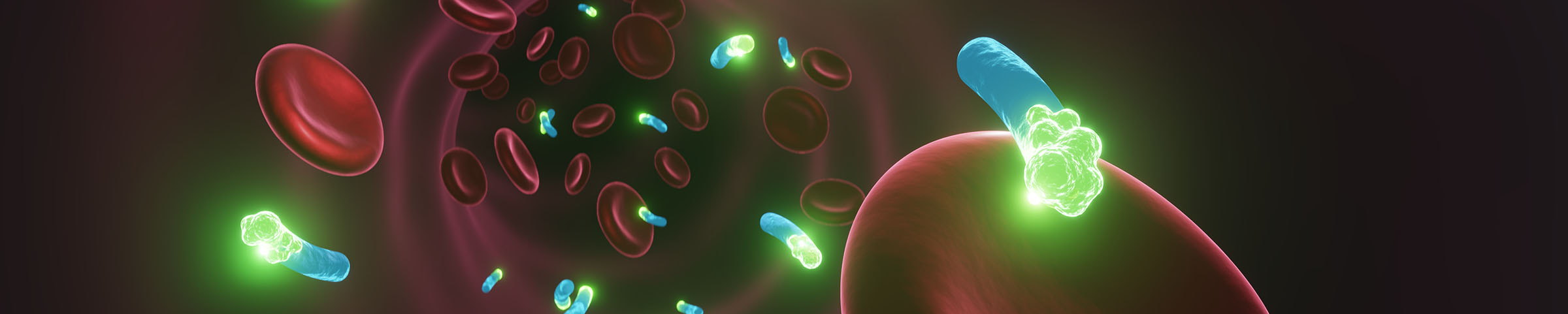
Kozimor leads a team of chemists, physicists, and bioscientists in the development and testing of an extraordinarily accurate Y. pestis antibody, attached to a few ions of actinium-225 via chelators, in a configuration that is therapeutically effective. Kozimor has been working through a series of increasingly effective chelators—and chelator design and analysis tools—while his Los Alamos colleague Armand Dichosa leads the biological efforts, including those by Lab scientist Antonietta Lillo to create and screen new antibodies for increasing specificity to Y. pestis—even when radiolabeled—while still maintaining sufficiently broad coverage across different Y. pestis strains. Meanwhile, other colleagues at Los Alamos and Lawrence Berkeley national laboratories have begun testing different aspects of the treatment.
At Los Alamos, scientists Jennifer Harris and Laura Lilley have been examining the effectiveness of radioisotope treatment for Y. pestis infections in human lung tissue, where the pathogen can cause pneumonic plague (as contrasted with bubonic plague, in which the pathogen is introduced through the skin, as from a flea bite). With pneumonic plague, antibiotic treatments are effective only if begun within 24 hours of the onset of symptoms, after which a radioisotope therapy could be a lifesaver. Harris and Lilley applied antibodies and isotopes to a suite of lung cells of different kinds, such as those involved in protecting the airway or oxygenating the blood. Their findings to date are quite promising.
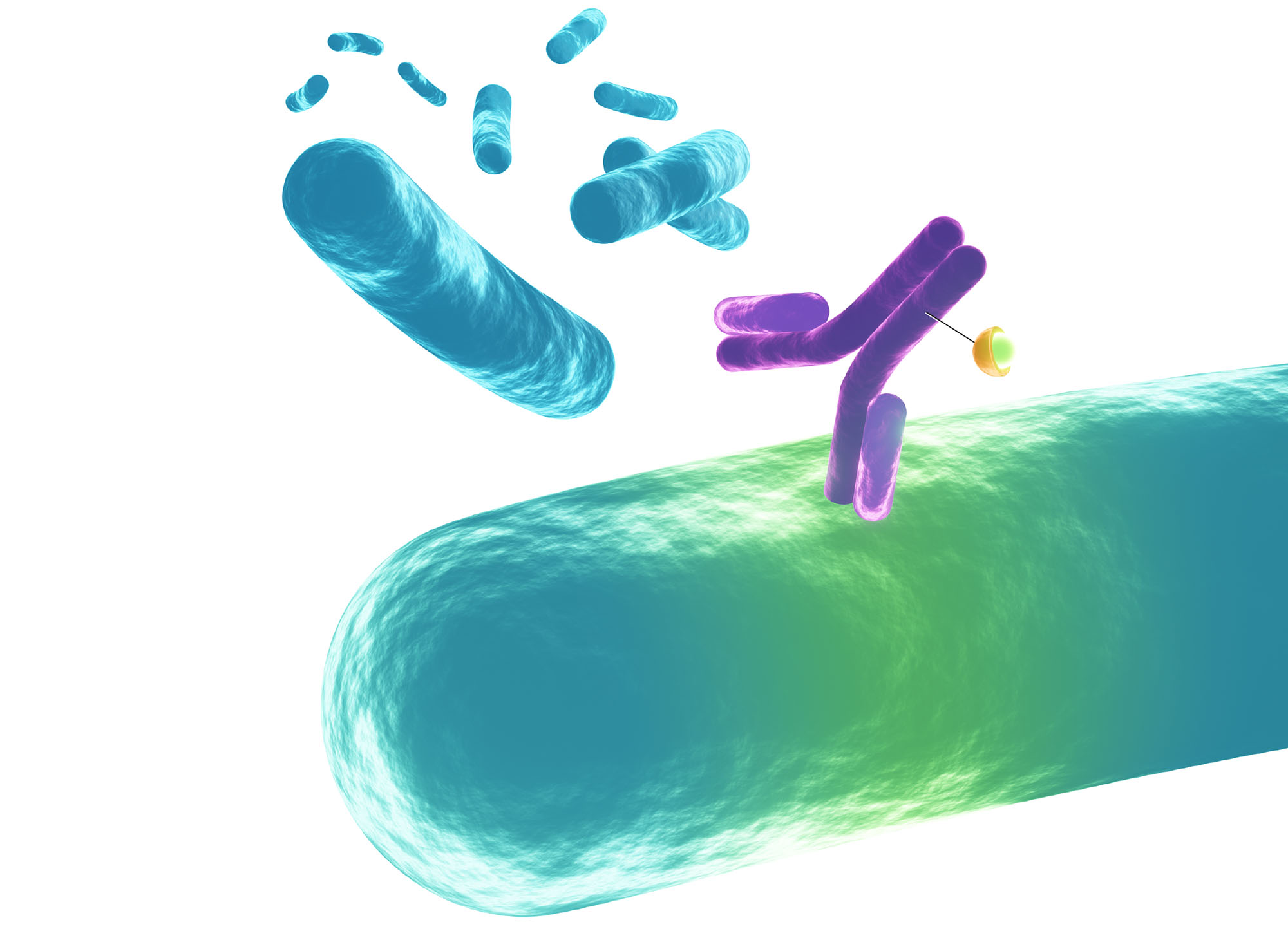
To find and kill dangerous pathogens (blue) within the body, an antibody (purple) that selectively binds to an antigen on the surface of the pathogen is linked to a chelator molecule (orange) designed to carry ions of a radioactive isotope with a brief half-life. Concentrated radiation from the attached isotope delivers a lethal dose to the pathogen before the isotope’s radioactivity safely dwindles away.
“First off,” says Harris, “our antibodies were not harmful to the lung tissues, which is reassuring, given that some antibodies can cross-react with tissue and damage it. But more than that, at radiation levels necessary to kill bacteria or stunt its growth, we found that mature, differentiated tissues—as found in actual human lungs—were much more stable after isotope exposure than the immature cells more commonly used in research. I consider this a serious win.”
The range of expertise needed to bring something like this together is truly expansive.
Meanwhile, at the University of California, Berkeley, and Lawrence Berkeley National Laboratory, collaborator and professor Rebecca Abergel is testing the treatment paradigm on mice, as well as in the petri dish. She is working with the whole package—Los Alamos’s targeted Y. pestis antibodies chelated with actinium-225—and preliminary results are extraordinarily encouraging. The petri-dish experiments on bacterial cultures reveal that delivering the actinium via antibody greatly diminishes bacterial survival, and the experiments in mice show reduced bacterial colonization in major organs. Much remains to be done to see if this approach will be safe and effective in human beings and, if so, to develop it into a properly sanctioned medical treatment. But as initial indicators go, these present about as much success as could be hoped for.
“This is a real chance to save lives, against a backdrop of increasing rates of drug-resistant bacterial infections,” says Kozimor. “But the range of expertise needed to bring something like this together—nuclear physics and manufacturing isotopes, exquisite antibody design, chelation chemistry, biomedical testing—is truly expansive. Where do you get this kind of varied expertise all in one place for the sake of safeguarding lives? To my thinking, this is exactly what our national labs are for.”