Los Alamos, N.M., Oct. 27, 2021—New results from a more-than-decade long physics experiment offer insight into unexplained electron-like events found in previous experiments. Results of the MicroBooNE experiment, while not confirming the existence of a proposed new particle, the sterile neutrino, provide a path forward to explore physics beyond the Standard Model, the theory of the fundamental forces of nature and elementary particles.
“The results so far from MicroBooNE make the explanation for the MiniBooNE experiment’s anomalous electron-like events more likely to be physics beyond the Standard Model,” said William Louis, physicist at Los Alamos National Laboratory and a member of the MicroBooNE collaboration. “What exactly the new physics is – that remains to be seen.”
The MicroBooNE experiment at the U.S. Department of Energy’s Fermi National Accelerator Laboratory explores a striking anomaly in particle beam experimentation first uncovered by researchers at Los Alamos National Laboratory. In the 1990s, the Liquid Scintillator Neutrino Detector experiment at the Laboratory saw more electron-like events than expected, compared to Standard Model-based calculations.
In 2002, the follow-up MiniBooNE experiment at Fermilab began gathering data to investigate the LSND result in more detail. MiniBooNE scientists also saw more electron-like events than calculations based on the Standard Model prediction. But the MiniBooNE detector had a particular limitation: It was unable to tell the difference between electrons and photons (particles of light) close to where the neutrino interacted.
The MicroBooNE experiment seeks to explore the source of the anomaly of the extra events. The MicroBooNE detector is built on state-of-the-art techniques and technology, using special light sensors and more than 8,000 painstakingly attached wires to capture particle tracks. It’s housed in a 40-foot-long cylindrical container filled with 170 tons of pure liquid argon. Neutrinos bump into the dense, transparent liquid, releasing additional particles that the electronics can record. The resulting pictures show detailed particle paths and, crucially, distinguish electrons from photons.
“Liquid argon technology is a relative newcomer to neutrino physics, and MicroBooNE has been a trailblazer for this technology, demonstrating what incredible physics one can do with it,” said Sowjanya Gollapinni, Laboratory physicist and a co-leader in the analysis. “We had to develop all the tools and techniques from scratch, including how to process the signal, how to reconstruct it, and how to conduct calibration, among others.”
MicroBooNE included a series of measurements: a photon measurement, and three electron measurements. In early October, results from the photon measurement, which specifically looked for Delta radiative decay, provided the first direct evidence disfavoring an excess of neutrino interactions due to this anomalous single-photon production as the explanation for the MiniBooNE energy excess. Delta radiative decay was the only background the MiniBooNE experiment couldn’t directly constrain.
The three new electron analyses address the question of whether the excess is due to an electron neutrino scattering on an argon nucleus, producing an outgoing electron. The new results disfavor that process as an explanation of the MiniBooNE excess, leaving the question of what causes the MiniBooNE anomaly still unanswered.
“In my mind, that neither photon nor electron production accounts for the excess makes understanding the MiniBooNE results more interesting, and more likely to venture into some very interesting physics beyond the Standard Model,” Louis said.
With only half the data from MicroBooNE yet assessed, possible explanations still to be considered (or tested in future experiments) include the possibility that still-unproven sterile neutrinos might be decaying into gamma rays. Axion decay – the axion is another hypothetical elementary particle – into gamma or an electron-positron pair might also be responsible. Sterile neutrinos and axions could be linked to the dark sector, the hypothetical realm of different yet-unobserved physics and particles.
“The possibilities are endless,” Gollapinni said, “and MicroBooNE will be on a mission to explore each of these with the full dataset. The results provide a pathway for further experimental physics, but a full understanding of the results will also depend on our theoretical physics colleagues, who are very intrigued by these results.”
MicroBooNE is one of a suite of neutrino experiments searching for answers. The ICARUS detector is beginning to gather physics data, and the Short-Baseline Near Detector (SBND) is coming online in 2023; both detectors use liquid argon technology. Together with MicroBooNE, the three experiments form the Short-Baseline Neutrino Program at Fermilab and will produce a wealth of neutrino data. For example, in one month, SBND will record more data than MicroBooNE collected in two years. Today’s results from MicroBooNE will help guide some of the research in the trio’s broad portfolio.
Further building on the techniques and technology of MicroBooNE, liquid argon will also be used in the Deep Underground Neutrino Experiment (DUNE), a flagship international experiment hosted by Fermilab that already has more than 1,000 researchers from over 30 countries. DUNE will study oscillations by sending neutrinos 1,300 km (800 miles) through the earth to detectors at the mile-deep Sanford Underground Research Facility, in South Dakota. The combination of short- and long-distance neutrino experiments will give researchers insights into the workings of these fundamental particles.
At Fermilab or underground in South Dakota, Laboratory researchers are contributing the technology and analytical understanding to probe the mysteries of particle physics. What lies ahead is unknown, but exciting.
“What we’ve found and continue to find with MicroBooNE will have significant implications for future experiments,” said Gollapinni. “These results are pointing us to a new direction and telling us to think outside the box. MicroBooNE’s journey to explore the exciting physics ahead of us has just begun, and there is much more MicroBooNE will reveal in the coming years.”
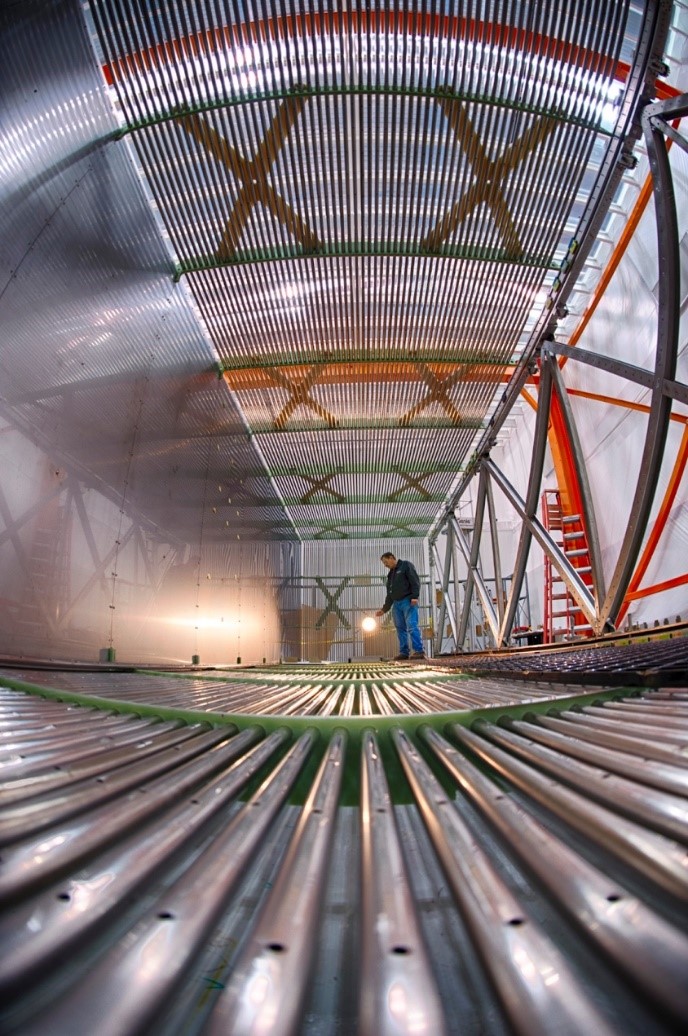
MicroBooNE is supported by the U.S. Department of Energy, U.S. National Science Foundation, Swiss National Science Foundation, U.K. Science and Technology Facilities Council, U.K. Royal Society, and European Union’s Horizon 2020.
About Los Alamos National Laboratory
Los Alamos National Laboratory, a multidisciplinary research institution engaged in strategic science on behalf of national security, is managed by Triad, a public service oriented, national security science organization equally owned by its three founding members: Battelle Memorial Institute (Battelle), the Texas A&M University System (TAMUS), and the Regents of the University of California (UC) for the Department of Energy’s National Nuclear Security Administration.
Los Alamos enhances national security by ensuring the safety and reliability of the U.S. nuclear stockpile, developing technologies to reduce threats from weapons of mass destruction, and solving problems related to energy, environment, infrastructure, health, and global security concerns.
LA-UR-21-30697