You may not consider americium-241 to be a household name. However, it is the one actinide that is found in almost every household: as an isotope that produces ionizing radiation, it is an essential component of smoke detectors. There are dozens of other important uses for americium-241, yet its supply as a controlled material has been unreliable—subject to international markets and associated geopolitical turbulence due to the governmental nature of international nuclear production sites. The US has been reliant on these unreliable supply chains since 2004, however recent efforts have reestablished domestic production of americium-241, which resumed in 2017 after a 33-year hiatus. This milestone has proved to be a turning point in the critical area of isotopes, eliminating a vulnerability from our radioactive source supply chain and reducing our dependence on sensitive sources.
Today, Los Alamos is proud to be the only manufacturing site of americium-241 in the US, having delivered its first shipment to customers in 2020. As an unwanted impurity that grows into nuclear waste streams and stockpiles of plutonium, americium-241 increases the radiation dose of these aging materials and makes downstream processing a challenge. Removal of this contaminant reduces the radioactivity of these waste streams and transforms a highly radioactive waste constituent into a valuable industrial and research product—a true win-win for both scientists and taxpayers.
In this article, we will examine how Los Alamos has achieved this milestone and also cover the history of americium, from the curious announcement of its discovery on a children’s radio show to its manifold uses that range from the commonplace to the celestial.
Quiz kids: Americium history
Americium was first isolated and characterized by Glenn Seaborg, Leon (Tom) Morgan, Ralph James, and Albert Ghiorso (Fig. 2) in late 1944 following experimental work at UC Berkeley, Clinton Engineer Works, and the Hanford Site, and analytical work at the Metallurgical Laboratory of the University of Chicago. Using the same 60-inch cyclotron that was employed to synthesize plutonium (1940) and curium (1944), they bombarded plutonium-239 with neutrons in small-scale reactions. Two successive neutron capture (n,γ) reactions occur, giving plutonium-241 (half-life of 14.3 years), followed by beta decay, yielding americium-241 (Fig. 1).
The details of the science were very subtle, prompting Seaborg to remark in a retrospective about americium and curium, “The understanding and interpretation of the results took place over a longer period of time and had more of the ingredients of a detective story than was the case for the other elements in whose discovery I had the privilege to participate.” If you want to read more about this particular “detective story,” you can find it in The Transuranium People: The Inside Story (2000) by Seaborg, Ghiorso, and Darleane Hoffman.

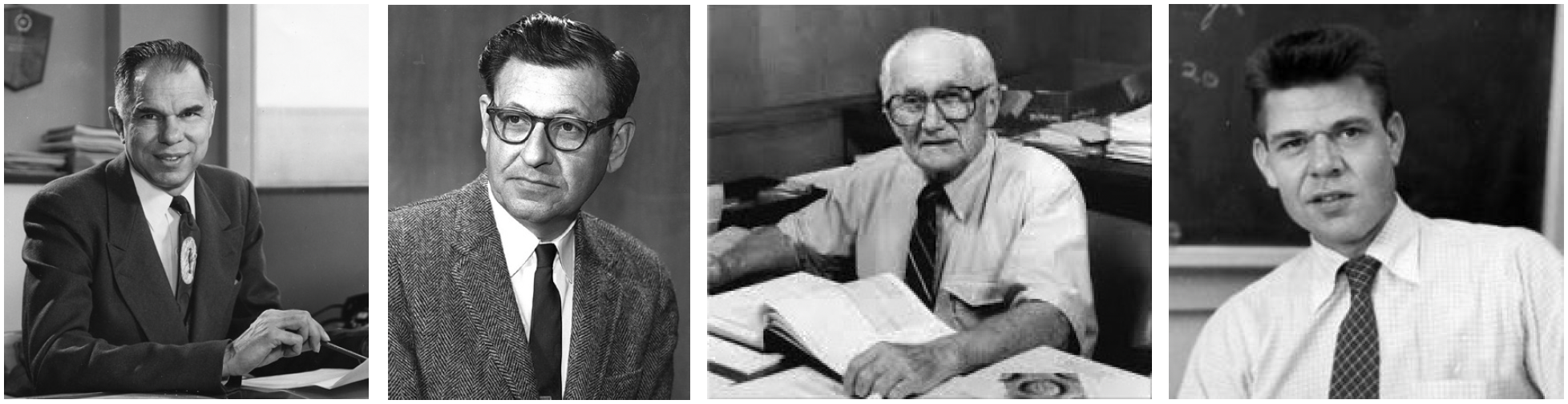
Courtesy: Lawrence Berkeley National Laboratory, courtesy of AIP Emilio Segrè Visual Archives
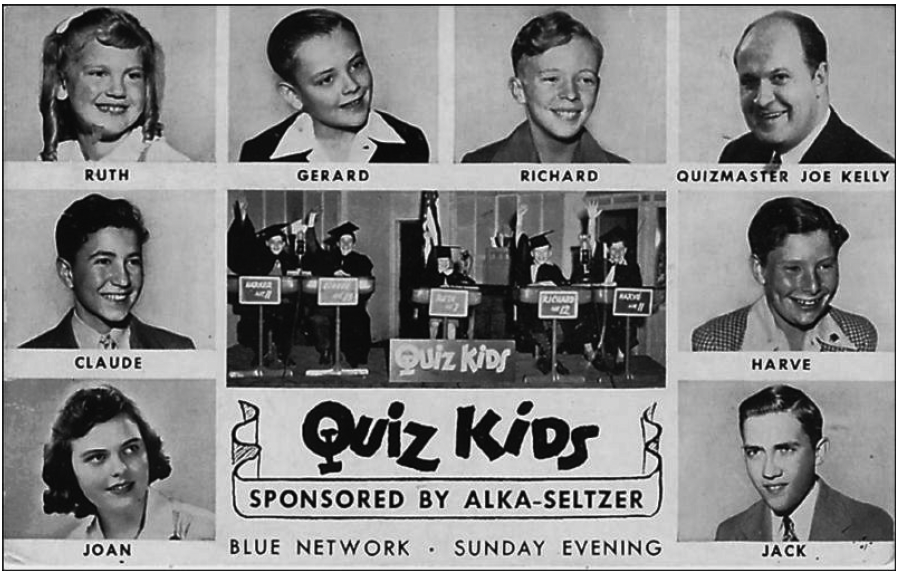
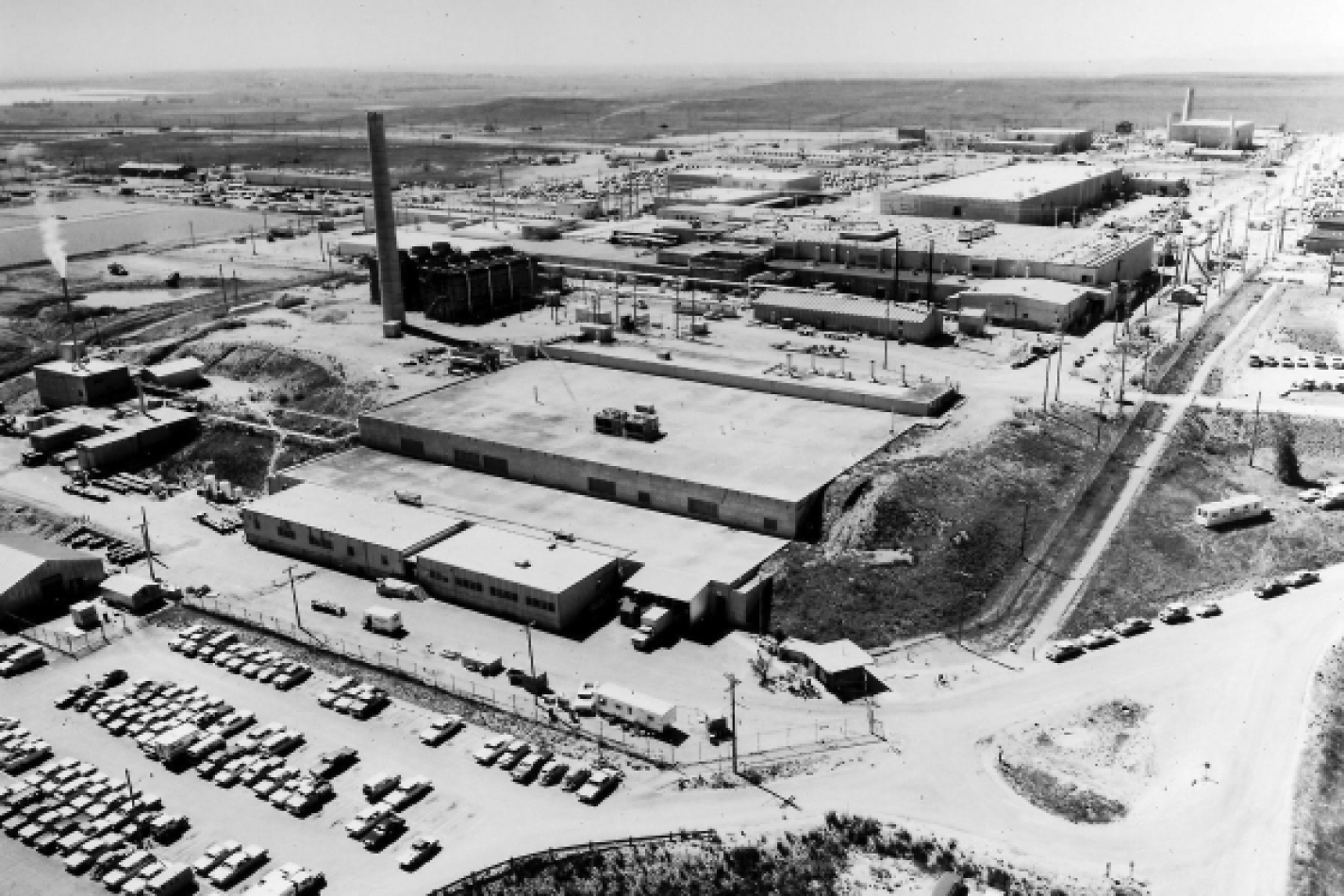
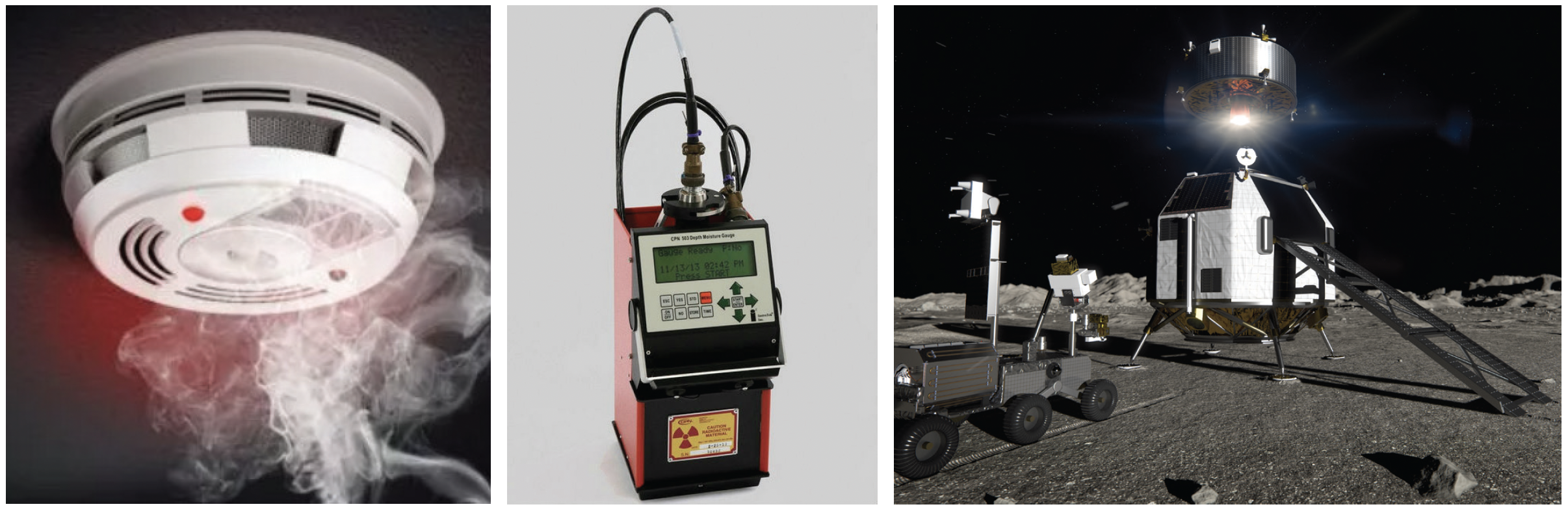
Figure 5. Americium-241 finds applications in smoke detectors (left), neutron moisture meters (center), and radioisotope thermoelectric generators (RTGs) for space exploration. Right: Artist's impression of a European Space Agency (ESA) Argonaut mission on the Moon. These missions, scheduled for launch in the 2030s, will use an americium-241-powered RTG to eliminate the need for solar panels and allow exploration of regions with little or intermittent sunlight.
Courtesy: ESA.
The announcement of these wartime discoveries came in an unorthodox manner the following November (1945) when Seaborg was invited as a guest scientist on the children’s radio show Quiz Kids. One of the children asked whether any new elements other than plutonium and neptunium had been discovered at the Metallurgical Laboratory in Chicago during the war. Seaborg replied, “Oh yes, Dick. Recently there have been two elements discovered—elements with atomic numbers 95 and 96. So now you’ll have to tell your teachers to change the 92 elements in your schoolbook to 96 elements.” This announcement was unplanned, but Seaborg decided to answer Dick’s question because the information had already been declassified for an official presentation at an American Chemical Society symposium in five days’ time. By analogy with the naming of its rare earth homologue, europium, after Europe, element 95 was named americium after the Americas.
Americium-241 was first made commercially available in 1962 by the US Atomic Energy Commission, manufactured at the Rocky Flats Plant in Golden, Colorado as a byproduct of plutonium processing. Production ceased in 1984, and the pit production facility was closed in 1989. By 2004, the remaining inventory of americium was depleted, leaving the US dependent on Russian supplies.
Properties and hazards
Americium-241 has a half-life of 432.2 years, decaying primarily through alpha emission to give neptunium-237 (Fig. 6). The second most common mode of decay is spontaneous fission, giving fission products and gamma rays. As such, the isotope is an essentially monoenergetic, high-energy alpha emitter (5.4–5.5 MeV) and a source of soft gamma radiation (59.5 keV); it has a specific activity of 3.43 Ci/g. As an alpha emitter, it does not pose a significant radiological risk unless taken internally, however the gamma radiation creates a significant external dose. Gloveboxes that are equipped to process americium-241 must therefore be heavily shielded with lead or equivalent material.
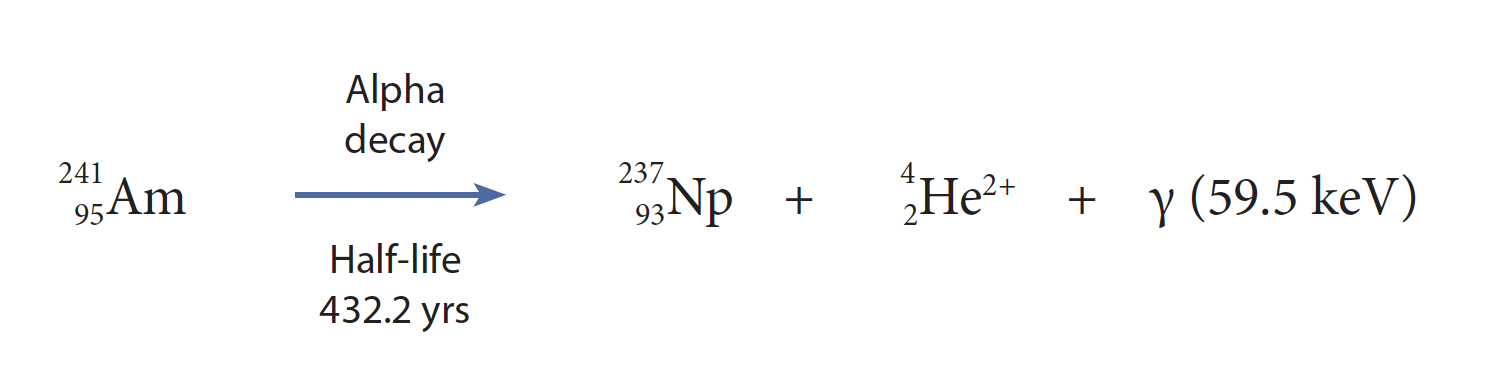
Americium-241 uses
Americium-241 is mainly used for its alpha emission, which is essentially monoenergetic and high energy (~5.4 MeV), although it has also been used in instruments as a source of soft (i.e., low penetrating) gamma rays. The most widespread use of its alpha radiation is as an ionization source in smoke detectors, where it is used in vanishingly small quantities—on a sub-microgram scale. Americium-241 is preferred over its alternative radium-226 because it emits five times more alpha particles and relatively little harmful gamma radiation at that scale. By ionizing the air, alpha radiation from americium enables an electric current to flow between two electrodes in a smoke detector—when smoke particles enter the detector, they block the current, and an alarm is sounded.
Another major use of americium-241 is as an indirect source of fast neutrons. Americium produces fast neutrons when paired with beryllium—the actinide acts as an alpha source in an (α,n) reaction. Beryllium is used because it possesses a large cross section for the (α,n) reaction, which then produces carbon-12 resulting from alpha particle capture (Eqn. 2, right). This is an extremely cheap and convenient source of fast neutrons compared to nuclear reactors or particle accelerators and can be used in hand-held diagnostic tools.
Fast neutrons have an important application in neutron moisture meters, which are among the most accurate devices available for measuring moisture content of soil (Fig. 5). By coupling a fast neutron source with a neutron detector, a device can provide an estimate of the amount of hydrogen present and therefore the amount of water. In the oil and gas industry, the Am-Be neutron probe is used in a similar fashion for well logging, i.e., creating a record (a well log) of the geologic formations penetrated by a borehole. In this type of neutron porosity log, the hydrogen atoms of hydrocarbons, both liquid and gas, are detected along with water. The porosity values describe how economically feasible the well could be by estimating the ease with which hydrocarbons will flow through the rock. The Am-Be neutron source is also used in imaging techniques such as neutron radiography and tomography, using fast neutrons in the place of X-rays.
As a gamma source, americium-241 has found use as an X-ray excitation source, yielding essentially monoenergetic X-rays whose energy can be tuned. Examples of these applications include materials analysis, using radiography and X-ray fluorescence spectroscopy, and nuclear densitometry in the civil construction, mining, and petroleum industries. The latter technique gauges a material’s density or thickness, for instance in soil and asphalt compaction tests for quality control or in industrial gauging where it is used to determine the thickness of plate glass, metals, and wire.
Americum-241 has been proposed as an alternative to plutonium-238 for use in radioisotope thermoelectric generators (RTGs), a type of nuclear battery that has no moving parts, used for unmanned applications such as space missions and remote lighthouses. In these applications, the power source is simply the heat generated by radioactive decay. In November 2022, the European Space Agency (ESA) approved funding for a program to develop americium-powered RTGs in the 10–50 watt range for a series of Moon missions planned for the early 2030s. In the past, the ESA has been reliant on US or Russian partners for supply of plutonium-238, but has since severed ties with Russia after the country invaded Ukraine in February 2022. Due to limited supplies of plutonium-238, they have chosen americium-241 as an alternative radioisotope for their RTG program on the grounds of availability and cost: it can be extracted by reprocessing used nuclear fuel and is five times cheaper per watt of power than plutonium-238. However, americium-241 produces less power per gram due to its longer half-life (432 versus 88 years). The ESA plans to use aged reactor-grade plutonium from the UK, originally containing about 10–14% plutonium-241 as precursor, to recover the americium-241.
DOE Isotope Program
The Department of Energy Isotope Program (DOE-IP) has its roots going back to the early days of isotope manufacture following the Manhattan Project. Today, the program manages the federal isotope industry, taking care of production and distribution of isotopes that are in short supply as well as maintaining infrastructure and conducting a portfolio of research and development that has been growing rapidly. The program has the sole authority within DOE to produce isotopes for sale and distribution (see www.isotopes.gov/catalog), with the exception of molybdenum-99, plutonium-238 for radioisotope thermoelectric generators, and special nuclear material produced for defense program purposes, which are handled by the National Nuclear Security Administration and the Office of Nuclear Energy. Money brought in from sales is used to reinvest in isotope production.
A key part of the Isotope Program mission is to reduce US dependency on foreign isotope supplies to ensure national preparedness. Through these efforts, which have substantially increased in the last decade, the program has now mitigated the dependence of many isotopes for which we previously relied on foreign countries. This includes americium-241. In 2009, the Isotope Program recognized the risks in the supply chain and provided support to a small team of researchers at Los Alamos to reestablish domestic production capability. Thanks to this funding, Los Alamos plutonium workers at TA-55 began work in 2017 to establish a new processing line at the Plutonium Facility (PF-4) to isolate americium-241 and convert it to an oxide for research and commercial sales.
Americium-241 recovery at TA-55
Americium-241 may be a desirable product in many industrial applications but at TA-55 it is an unwanted radioactive impurity that creates additional gamma radiation dose in aged plutonium materials, causing increased risk to workers and making downstream processing more complicated. It grows into nuclear waste streams and plutonium stockpiles at a rate that can be calculated by the quantity of plutonium-241 isotope originally present in the sample, along with its age. Plutonium-241 has a half-life of 14.3 years, producing americium-241 as its decay product at an approximate 5% annual rate (atom basis). Roughly speaking, this means that after 20 years, 1 kg of fresh weapons-grade plutonium will contain around 6 g of americium-241.
Virtually all plutonium operations at Los Alamos occur within PF-4, a 233,000 square-foot Hazard Category 2 defense nuclear facility. A significant proportion of this work involves chemical processing of plutonium metal or its compounds, such as plutonium dioxide (see Actinide Research Quarterly Third Quarter 2008 for more information). This includes aqueous chloride (AQCL) extraction activities, which operate as an alternative to aqueous nitrate (AQN) recovery lines.
The aqueous chloride mission is to recover plutonium and americium from pyrochemical residues, mostly chloride salts that are not appropriate for applications and/or storage. This extraction process generates plutonium oxide, which is suitable for storage, and americium oxide, now for sale through the DOE Isotope Program. The pyrochemical residues that act as feedstock for these processes come from high-temperature pyrochemical techniques used to purify aged plutonium metal or other plutonium sources (as described in the Los Alamos Pit Production Flowsheet, Fig. 12). A step in the process removes americium-241 ingrowth from the metal, giving an impure byproduct salt which becomes the feed for recovery of plutonium and americium along with legacy supplies.
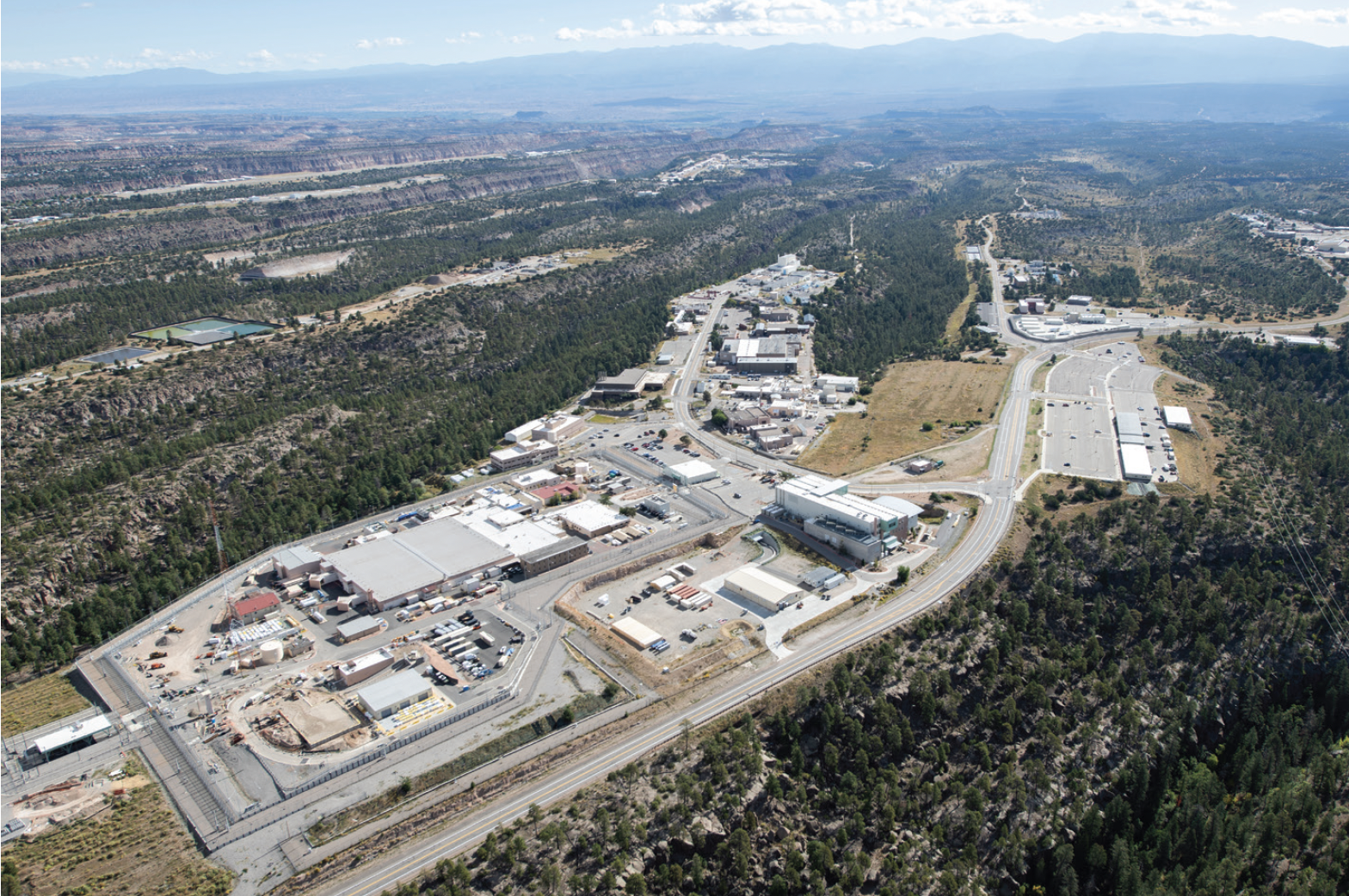
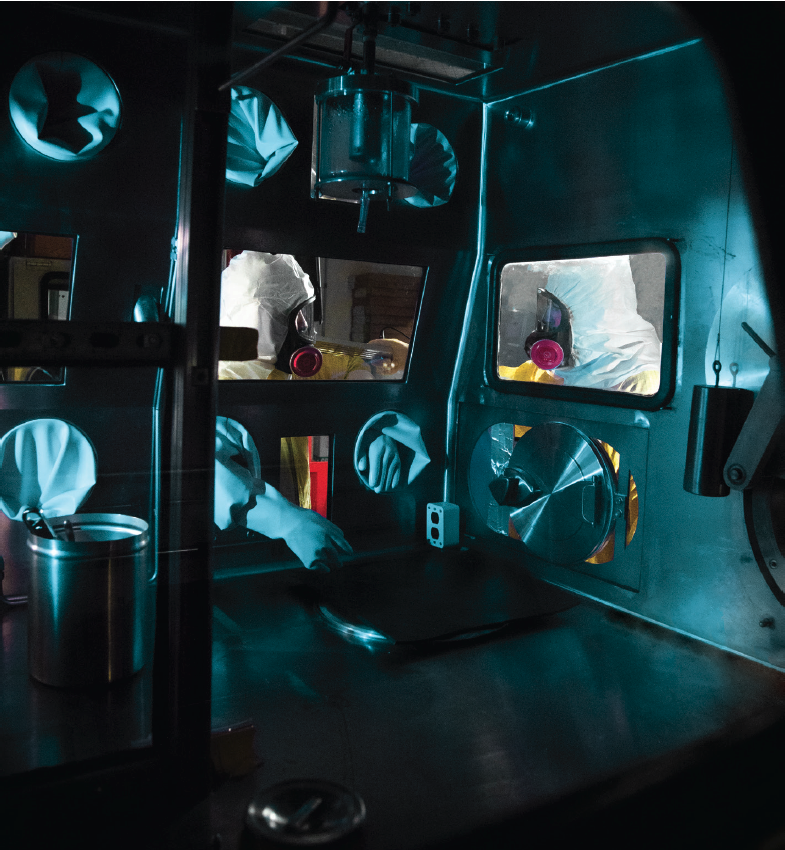
Recovery of plutonium and americium is achieved using two chemical extraction lines contained within radiological gloveboxes. The experimental chloride extraction line (EXCEL) has operated since 1993 to purify plutonium materials through aqueous chloride extraction. More recently in 2017, the chloride line extraction and actinide recovery (CLEAR) glovebox system was added downstream of EXCEL for americium-241 oxide recovery and purification. Extractions are typically performed on a multigram scale.
The EXCEL/CLEAR process consists of the following six main steps:
1. Dissolution. Plutonium solids (pyrochemical residues) are dissolved in aqueous hydrochloric acid, giving the primary species plutonium(III) chloride plus americium(III) chloride and other impurities.
2. Separation. Plutonium, americium, and impurities are separated using either solvent extraction (SX) or anion exchange (IX) methods. Two streams are created, one containing plutonium(IV) and the other americium(III).
3. Americium-241 purification. The first stage in the CLEAR process, using preparative-scale chromatography to extract americium from the waste stream created by the previous plutonium separation process.
4. Precipitation. Oxalate precipitation using oxalic acid is used in both streams:
- (i) Plutonium stream: Hydroxylamine hydrochloride is used as a reducing agent which converts the plutonium(IV) to plutonium(III). Oxalic acid is then added to precipitate plutonium oxalate.
- (ii) Americium stream: A reducing agent is not necessary here as the americium is already in the appropriate (trivalent) oxidation state. Oxalic acid is added to precipitate americium oxalate.
5. Calcination. Plutonium and americium oxalate cakes are calcined to create the respective tetravalent oxide products. Plutonium is calcined in a tube furnace, americium in a muffle furnace.
6. Hydroxide precipitation. This step isolates and reduces the volume of radioactive waste products for disposal. The waste product wash solutions from SX/IX streams (step 2) and filtrate from the plutonium and americium oxalate precipitation (step 4) are combined and neutralized with potassium hydroxide, resulting in hydroxide precipitation. These solids are then calcined as described above to produce solid waste for radioactive disposal and a discardable caustic stream.
Separation: Solvent extraction (SX) versus anion exchange (IX)
The EXCEL process can be performed with either solvent extraction (SX) or anion exchange (IX) separation methods. In solvent extraction, a biphasic aqueous-organic extraction method is used. Using a motorized set of cylindrical “contactors,” the acidic aqueous stream containing dissolved plutonium and americium contacts an organic phase and separates naturally by density. The organic phase extracts plutonium using tributylphosphate (TBP) in a diluent of dodecane and decanol, similar to the PUREX (plutonium uranium reduction extraction) process for recovery of plutonium and uranium from spent nuclear fuels. The contactors are specially designed vessels that mix and separate the phases, allowing the process to be automated with continuous flow. The system uses several washing steps and eventually re-extracts the plutonium into an acidic aqueous phase using a total of eight contactors. The americium meanwhile is retained in the produced liquid, or raffinate, along with impurities for separate purification in CLEAR.
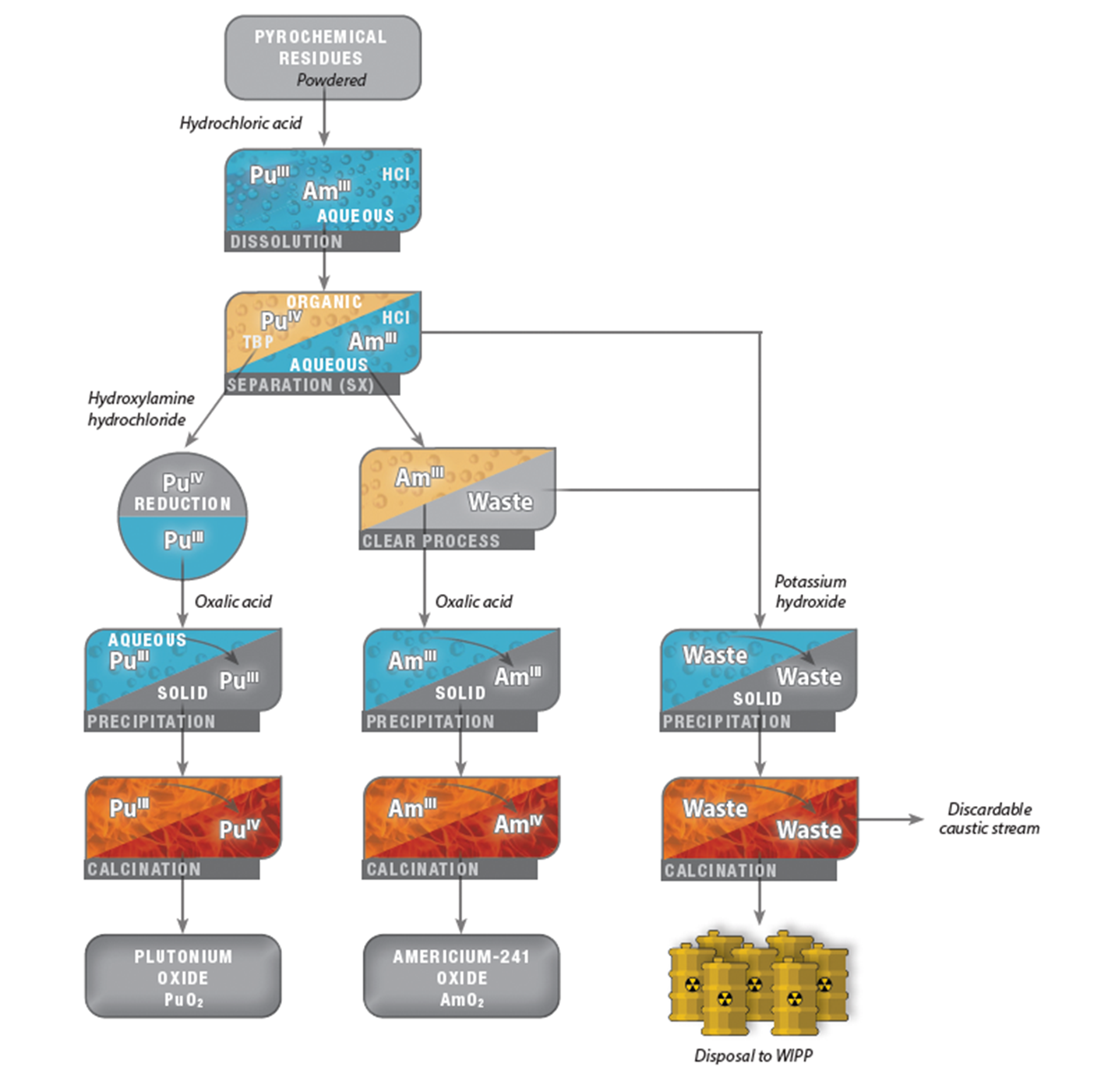
In the anion exchange process (IX), preparative-scale column chromatography is used in which plutonium and americium adsorb to different resins and are separated from waste products which are washed through the column. Once the actinides are washed, they are extracted using a low concentration HCl solution. Four columns are required using Reillex™ HPQ ion exchange resin, a material developed in the late 1980s through a collaboration between Los Alamos and private industry. Compared with earlier anion-exchange resins, HPQ has improved sorption properties for plutonium and is less prone to radiolytic or chemical degradation in harsh acidic conditions containing high concentrations of alpha-emitting plutonium isotopes. The enhanced stability of Reillex™ HPQ allows the resin to be used for approximately 50 plutonium recovery cycles before being replaced.
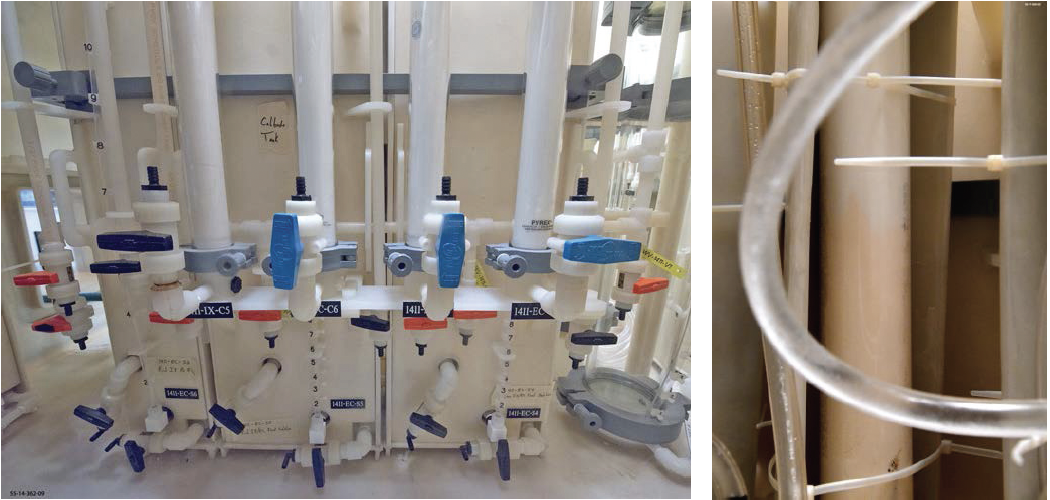
Despite being more labor-intensive, solvent extraction (SX) is currently the preferred system due to the better processing rate and higher capacity of nuclear material compared to anion exchange (IX). The latter requires running the process multiple times to purify a single batch, making it slower overall and producing more waste solution volume.
CLEAR: Extraction chromatography
The key step in the CLEAR process is extraction chromatography, similar to the anion exchange step. A LANL-developed phosphine oxide extractant (m-CMPO, di-(4-t-butylphenyl)-N,N-di-iso-butylcarbamoylmethylphosphine oxide) is used, which is adsorbed along with a phase transfer catalyst (TBP, or di-amyl amyl phosphate, DAAP) on a polymethacrylate resin bead. Recent research published by LANL researchers in 2021 suggests that changing from an m-CMPO-based resin to a commercially-available diglycolamide resin could make americium-241 recovery efforts more efficient. The diglycolamide resins quantitatively released americium-241 at low HCl concentrations (<0.5 M), were less susceptible to negative side effects from metal contaminants in the mobile phase, and bound americium-241 faster.
Americium oxide product
The stable form of americium-241 that is sold and used in instruments is the dioxide, AmO2, which is obtained as a beige-brown powder from the final two steps of the CLEAR process. The first of these steps transforms the chloride into a solid oxalate salt, which readily precipitates. This oxalate salt is then calcined in a furnace at high temperature to produce the oxide. This is performed on a much smaller scale than the calcination of plutonium because of the dose inherent with americium-241 and the amounts of daughter isotope present in the original material. Purity of the americium product is then ascertained using thermal ionization mass spectrometry (TIMS) to ensure it reaches minimum specifications (Table 1). Finally, the powder is blended for even particle distribution and packaged in a set of specially designed nested containers such as SAVY-4000, a vented, general-use nuclear material container.
Several sub- to multi-gram shipments of AmO2 product have been recovered by the Los Alamos americium-241 team since 2017 and made available for isotope sales through the DOE Isotope Program’s National Isotope Development Center (NIDC). The team that achieved this goal includes past and present members from AMPP-4,
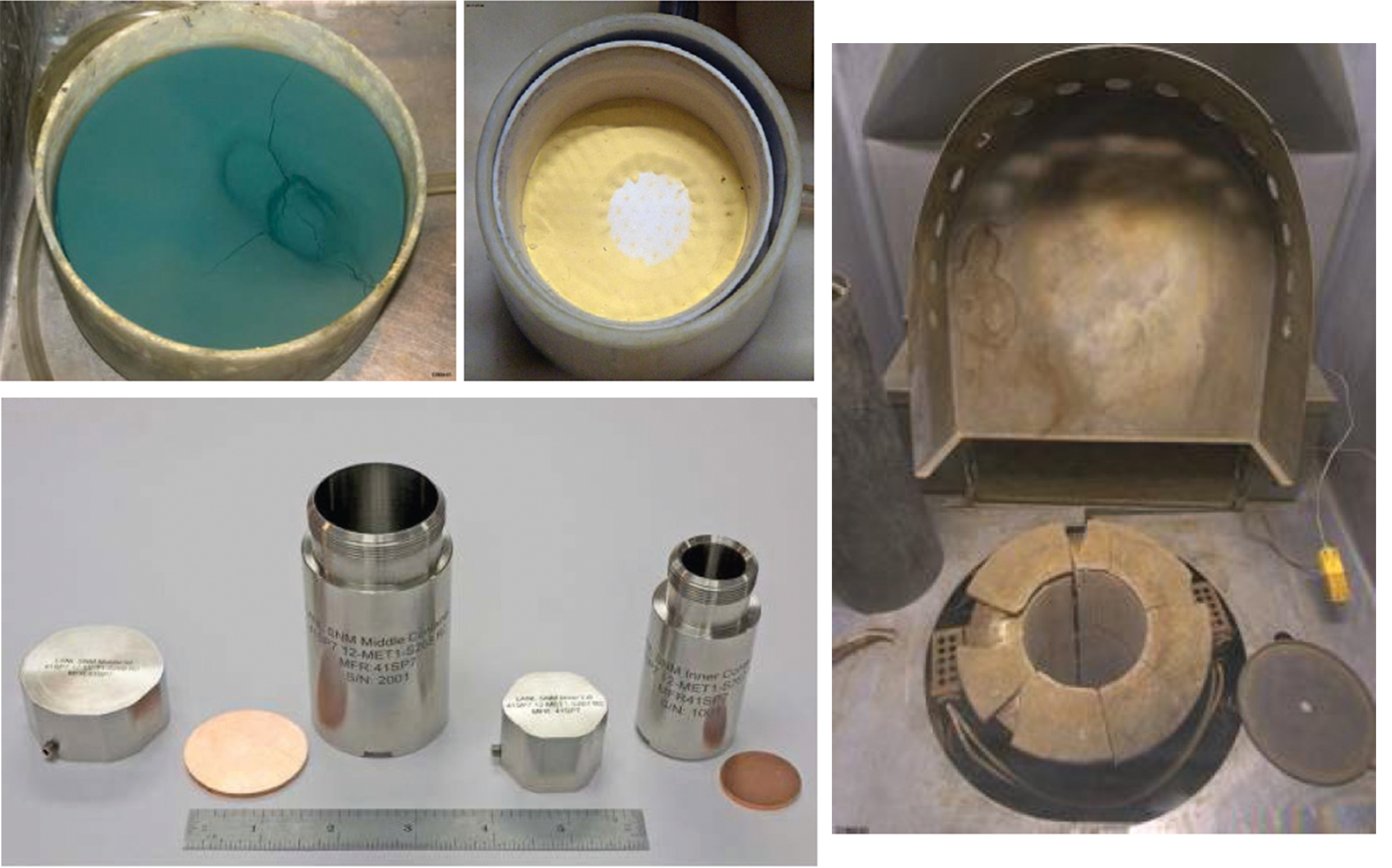
Figure 11. Left: The 241AmO2 product is packaged in a set of specially designed nested containers for transport.
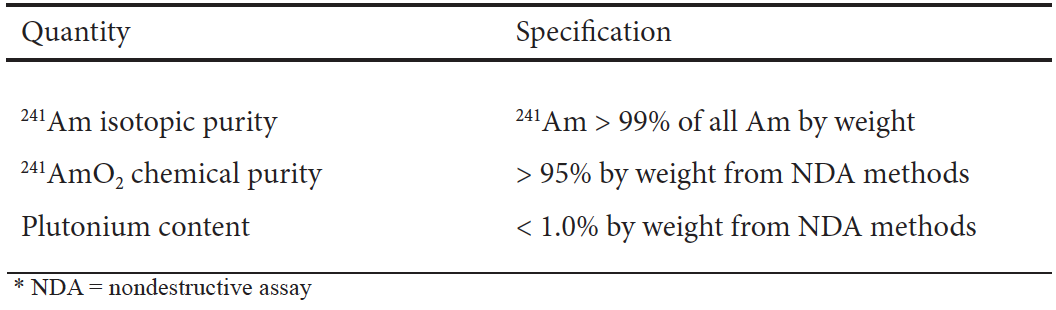
AMPP-3, ORI-2, and MST-16: Victor Salazar, Richard Salazar, Brenda Griego, David Kimball, Casey Finstad, Louis Schulte, Mary Ann Stroud, Brad Skidmore, Hope Quintana-Martinez, Gabrielle Ambrosio, Chris Lopez, Garrett Fulcher, Sheldon Apgar, and Andrew Wiggins.
Collaborations with other teams and organizations within LANL have dramatically improved several aspects of the CLEAR process. For instance, with the recent project described above that identified better extraction resins to increase the efficiency of americium-241 recovery. Since the inception of the CLEAR process, the aqueous chloride plutonium and americium recovery team has sought to increase yearly throughput of americium-241 oxide recovery and waste minimization. Successes with extraction resins and precipitation parameters are enabling this goal.
Interest in americium-241 recovery as part of the PF-4 pyrochemical flowsheet has increased with the 30 pits per year (30PPY) LANL mission. More pits create more
pyrochemistry, which entails processing more pyrochemical byproducts—all carrying significant quantities of americium-241. Ideally, to keep up with byproduct (waste) generation, EXCEL and CLEAR operations must become faster and more efficient.
Efforts are underway to streamline the CLEAR process and increase recovery of americium-241. The extraction chromatography process is still rate limiting in the CLEAR flowsheet, however improvements here have the potential to both speed up recovery rates and increase recovery amounts. Initial results using selective precipitations to purify the majority of the americium-241 show promise to achieve faster rates and larger volumes.
Summary
In 2009, the Department of Energy Isotope Program (DOE-IP) identified a vulnerability in the supply chain of americium-241 and recommended that domestic production resume from a Los Alamos plutonium waste stream. This isotope is in high demand, particularly in nuclear power sources, research, and as a portable source for medical and industrial applications. In 2012, DOE funded a small team of researchers at Los Alamos to reestablish a domestic production capability: workers at the Plutonium Facility (PF-4) consequently developed a new glovebox system: the chloride line extraction and actinide recovery (CLEAR) line. In 2017, operations began that gave the first delivered product of 241AmO2 in 2020, providing an efficient and effective domestic americium-241 supply that is critical for US research and industry. A secondary but substantial economic benefit also results in removing an unwanted impurity from plutonium residues, which would otherwise be costly to dispose. For instance, waste drums sent to WIPP (Waste Isolation Pilot Plant) in Carlsbad, New Mexico cost around $100,000 each. Recovery of plutonium and especially americium from the waste reduces its disposal footprint and saves on taxpayer’s dollars. Overall, resuming production of americium and mitigating dependency on sensitive countries has been beneficial to all parties involved: the US nuclear complex, taxpayers, and industry. A true win-win!
Further reading
1. B.T. Arko, D. Dan, S.L. Adelman, D.L. Huber, D.B. Kimball, S.A. Kozimor, M.N. Lam, V. Mocko, J.C. Shafer, B.W. Stein, S.L. Thiemann, “Characterizing extraction chromatography for large-scale americium-241 processing,” Ind. Eng. Chem. Res. 2021, 60, 14282.
2. D.C. Hoffman, A. Ghiorso, G.T. Seaborg, “The Transuranium People: The Inside Story,” Imperial College Press, London, 2000.
3. Actinide Research Quarterly, Third Quarter 2008.
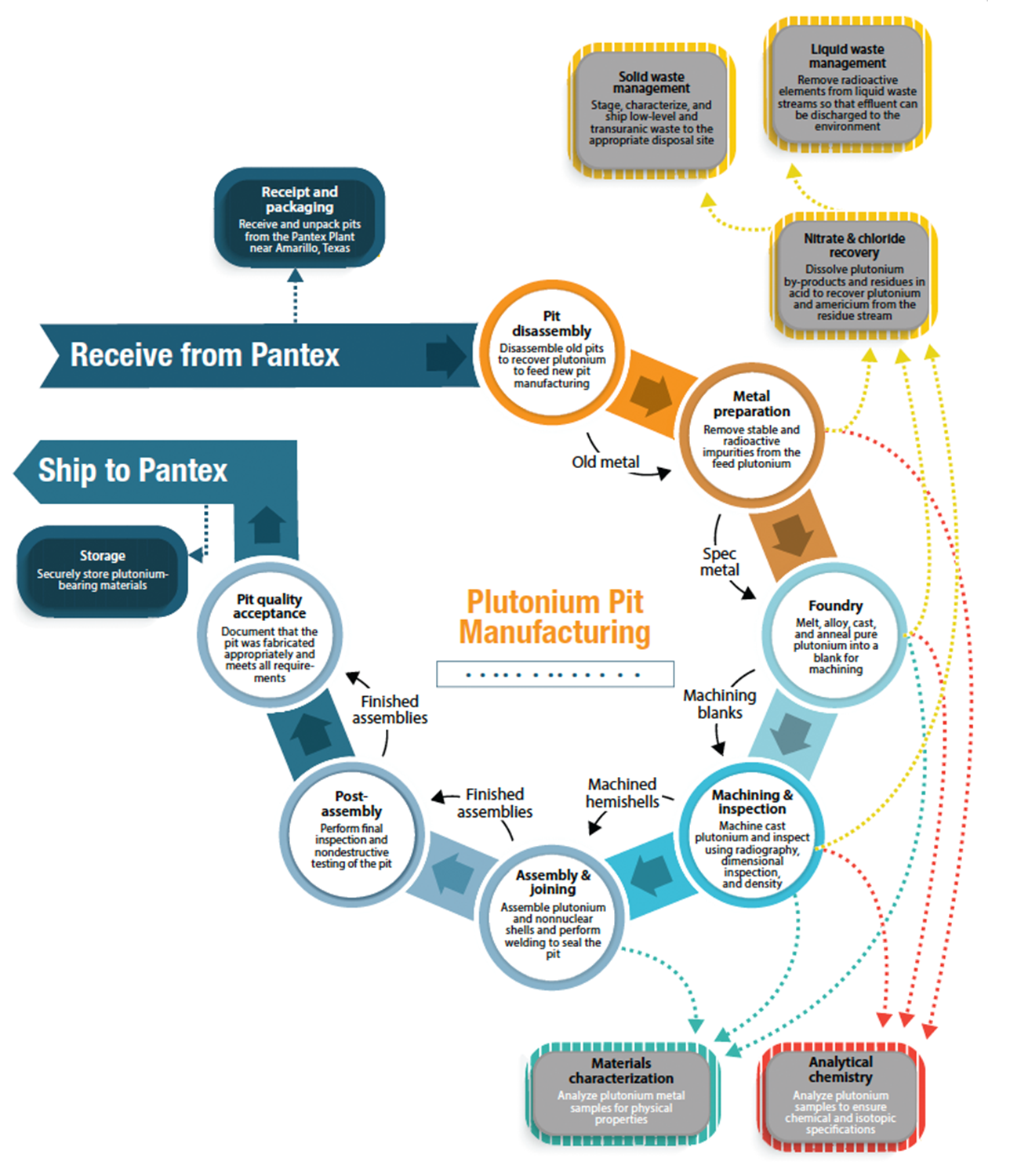
Courtesy: Brenda Fleming, National Security Science magazine.