By Katarzyna Krzyzanowska
While computers exploiting the exotic nature of quantum mechanics garner most of the press around quantum research, the related technology of quantum sensors deserves its share of attention. Particles and atoms in certain quantum states have the potential to make the most exquisite sensors for detecting phenomena such as magnetic fields and changes in inertia. Those abilities have a range of national security applications, which include inertial navigation systems that can still operate without a GPS signal, improved radar and LIDAR, underground mapping, and detection of underground explosions, to name a few.
Quantum sensors show great promise, but much basic science remains to realize their potential. Research at Los Alamos National Laboratory as part of the Quantum Science Center is drawing on the Lab’s leading-edge work with quantum algorithms. In a unique synergy between theory and experiment, the Laboratory team seeks to develop hardware that generates quantum correlations and provides a clear quantum advantage in sensing, going far beyond anything a conventional sensor can do.
Quantum cousins
As close cousins, quantum sensors and quantum computers have a lot in common. They both use quantum bits, or qubits, as their fundamental unit of information. Analogous to bits in classical computing, which can be a 0 or 1, qubits go further and can be in a state of quantum superposition, which is related to their probability of being a 0 or 1. That greatly expands their power compared to classical bits.
Both quantum computers and quantum sensing also rely on correlations among particles to process information or detect phenomena, respectively. Entanglement, the strongest form of correlation, describes the state of multiple particles sharing the same information; reading the state of one particle likewise reads all the others.
Quantum superpower
Harnessing that trait is at the core of quantum sensors’ superpower. A sensor can use qubits to establish any functional amount of quantum correlations, from a mixture of quantum and classical states up to a full entanglement. These states allow you to gain information about an entire correlated quantum system from the tiniest piece of it.
Suppose you have a single atom in its ground state, or lowest energy state, and you create correlations with many other atoms. Exposing that first atom to, say, an electromagnetic field will raise the atom to its excited state—and all its correlated kin will “read” that new excited state. In other words, the disturbance of its quantum state can be read from the correlated particles.
If you expose all the atoms to the external field, they will all individually get excited and also read the disturbance of each other. This way the sensitivity of the entire system is greater than using the same amount of uncorrelated particles where each atom interacts with the external field individually without sharing it with others.
Quantum sensing works well in theory, but is devilishly difficult in practice. It shares challenges with quantum computing, including susceptibility to noise in the form of interference from the surrounding environment. That interference weakens the correlations or even destroys the “quantumness” of the system, collapsing the superposition, at which point it follows the laws of classical physics rather than the rules of quantum mechanics.
Recipe for success
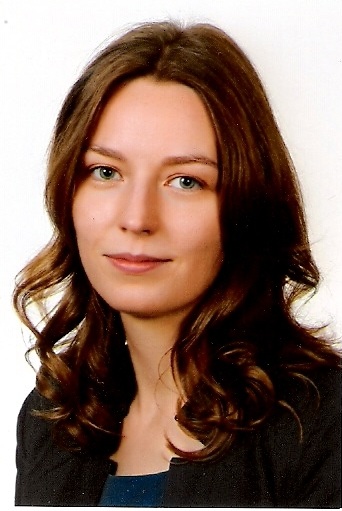
To compensate for the crippling disruption caused by noise in quantum computing, theorists at Los Alamos are developing variational quantum algorithms (VQAs) to probe the noise of a given quantum computer. VQAs issue a set of instructions to prepare a desired quantum state among the qubits in the computer. The VQA runs many times, training itself to find the best states that will yield quantum advantage, based on the computer’s unique noise profile. The resulting recipe tunes the qubits to the noise of that computer.
A similar approach translates to laboratory experiments for preparing a quantum sensor by manipulating atoms with a laser, for instance. In one such vein, our team at Los Alamos implements a lattice of single atoms, then applies a gate to engineer specific correlations between the atoms on the lattice and neighboring atoms. In another vein, we establish correlations between a single atom and many particles in a Bose Einstein condensate. The condensate is essentially an aggregate of supercooled particles that share a quantum state at a larger, macroscopic scale.
With these approaches, developed with our theory collaborators, we hope to develop sensors with quantum advantage, detecting phenomena with a sensitivity far beyond that of classical-physics sensors. Armed with these amazing probes, scientists in a range of fields will be able to investigate the world in amazingly fine-grained detail. Exciting new discoveries will surely follow, along with practical applications in the national security arena and far beyond, from more powerful MRIs to submarines and drones.
Katarzyna Krzyzanowska leads the team at Los Alamos National Laboratory researching quantum sensing as part of the Quantum Science Center, which is led by Oak Ridge National Laboratory.
LA-UR-21-31025 Version 2