The concept of using radioactive isotopes for medical applications has become commonplace in the last several decades.
Each day, tens of thousands of medical procedures across the globe use isotopes such as fluorine-19, technetium-99m, and iodine-123 and -124, coupled with positron emission tomography (PET) and single photon emission computed tomography (SPECT), to image the human body and diagnose diseases. In addition to these diagnostic imaging technologies, the use of targeted radiation can treat diseases. The strategy of infusing radioactive isotopes into the body presents some considerable advantages compared to conventional therapeutics, like those used in chemotherapy. These benefits include potency, reduced off-target side effects, reduced drug resistance, and the potential for personalized medicine. Significant technical challenges must be overcome before these benefits can be realized, however. One major challenge is controlling where an isotope is delivered within the body. Typically, this involves a multicomponent construct that holds the radioisotope with strong chemical bonds (chelator) and delivers it to diseased cells using a biologically active molecule that seeks the specific diseased tissue type (targeting vector). A cartoon representation of this system is shown in Fig. 1, where an antibody represents the targeting vector and representative examples of chelators (ligands that form two or more bonds to a single metal cation) are included.
Even when using an isotope of an element with well-known biochemical behavior, this construct is difficult to achieve. With some of the most promising radioisotopes, including several actinide isotopes, the challenge is even more significant. This is certainly the case for one of the most exciting candidates for targeted alpha therapeutics (TATs), actinium-225 (225Ac). Several of the fundamental nuclear properties of 225Ac make it ideal for clinical application including: a half-life of 9.920(3) days, four successive alpha decays, and the high energy of these alpha emissions (5–6 MeV). However, actinium chemistry is in a state of relative infancy compared to many other elements on the periodic table due to its radioactivity and associated challenges. Aqueous chemistry characterization of actinium is sparse, making it difficult to target/design molecules to bind 225Ac in stable complexes for infusions. The high dose rates, inherent from the short half-life of 225Ac and its daughters, pose significant challenges in handling. The high dose rate also mandates that relatively small quantities are handled at one time, which precludes characterization via macroscopic techniques. Logically, this would lead to characterization by nuclear spectroscopy, where intensity correlates to decay rates rather than mass alone. In the case of 225Ac, however, no convenient γ-emissions characterize the isotope directly and α-spectroscopy gives a convoluted overlapping spectrum in which daughter isotope α-emissions interfere with meaningful analyses.
Even if large amounts of this isotope could be safely handled, they are not readily available. 225Ac is in limited supply and typically comes from 229Th decay or is produced directly in high energy accelerators, creating an issue of accessibility.
Collectively, these problems make the complex technical challenges that stymie development of 225Ac therapeutics even harder to solve. Our approach to advancing the progress of 225Ac-based alpha therapeutics has focused on solving the problems listed above. Using this strategy, we hope to make R&D tools available to the greater community of actinium researchers to advance the development of this potentially game-changing therapeutic agent.
Actinium-228 as a spectroscopic tool: An underutilized surrogate for clinically relevant isotopes
Natural thorium (232Th, t½ = 14 billion years) slowly decays to 228Ac (t½ = 6.14(8) hours). The decay of this short-lived actinium isotope to 228Th(β–) releases unique and high intensity gamma emissions, making it very easy to characterize using routine nuclear spectroscopy. Therefore, 228Ac can be utilized as a surrogate isotope for the clinically significant 225Ac. The decay scheme and γ spectrum of 228Ac are shown in Fig. 3.
The main obstacle to implementing 228Ac as a radiotracer for 225Ac was the issue of harvesting minuscule amounts of 228Ac’s direct parent, 228Ra, from bulk 232Th (nanograms of 228Ra are generated in tens of grams of 232Th over many years). Isolating the parent 228Ra creates an 228Ac generator. This generator facilitates repeated ingrowth of the daughter (228Ac) in the parent solution (228Ra). The daughter isotope can then be repeatedly harvested and used for additional experiments by separating the parent and daughter isotopes. This method precludes harvesting the daughter directly using a single-pass method, such as harvesting 228Ac directly from 232Th, which only provides access to the isotope one time.
To read the full article, click here
Meet The Writer
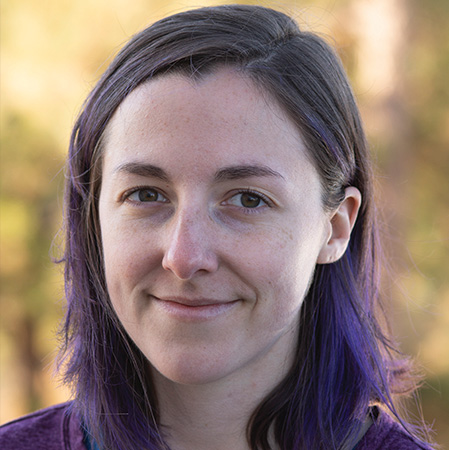