Los Alamos scientist Chris Yeager has spent his career examining microorganisms’ role in recycling the elements of life—decomposing dead plants and animals and releasing nutrients into the soil and atmosphere to help new life thrive. Ten years ago, while working at the Savannah River National Laboratory (SRNL), Yeager joined a team that was studying radioactive elements in soil and groundwater contaminated by nuclear waste. As the only microbiologist on the team, Yeager brought expertise that could help determine whether or not microorganisms play a role in the behavior of certain elements in the waste, including the “hot” ones. But even he was surprised by what they discovered.
“All of our research tells us that microbes are one of the principal actors determining what happens to radioactive particles in the soil,” says Yeager. Although he moved to Los Alamos in 2011, the implication of microbial involvement has kept Yeager closely tied to the soil contamination project ever since. “We have built a conceptual model showing microbes’ essential role in controlling the fate and transport of select radioactive elements. This information is critical for understanding the spread of contamination through soils and sediments.”
And if microbes are impacting the spread of contamination, then perhaps they can be used to control it. Microbes often recycle nature’s messes, but it is not unusual for them to recycle man-made ones as well. This is because microbes are ubiquitous, having adapted to live everywhere, including extreme environments that don’t appear to be very habitable. For instance, some microbes have evolved a propensity for metabolizing crude oil, making them valuable partners for cleaning up oil spills.
Yeager and his colleagues studied many sites contaminated by two nuclear waste products, radioactive iodine and plutonium, with the expectation that their findings could improve hazard assessments. In doing so, they learned a great deal about what happens to radioactive elements when they interact with microbes in the environment. And what they discovered now stands to significantly change how the U.S. Department of Energy (DOE) develops future models of radioactive contaminant mobility—which in turn could influence how the department approaches cleanup efforts.
Iodine: friend and foe
Iodine is an essential dietary nutrient that can participate in both causing and curing disease. It is required by the thyroid gland for the production of hormones that regulate metabolism and growth in the body. Because the thyroid absorbs iodine for this purpose, accumulation of a dangerous version, such as the radioactive isotope iodine-131, poses a serious cancer risk.
Radioactive isotopes iodine-131 and iodine-129 are byproducts of nuclear fission reactions. They can be found in waste from nuclear power plants and are released into the atmosphere by the detonation of a nuclear weapon. In the case of people exposed to radiation during large-scale nuclear accidents, such as those that occurred at the Chernobyl and Fukushima power plants, the deliberate consumption of more iodine can actually help mitigate the damage—like fighting fire with fire. Nonradioactive potassium iodide is given to people who may be exposed to radiation in order to saturate their thyroid glands, preventing the radioactive iodine from binding and causing disease.
Microbes are one of the principal actors determining what happens to radioactive particles in the soil.
In a starkly different situation, where thyroid cancer is already apparent, iodine‑131 is actually used to treat the cancer because it naturally targets the thyroid gland, delivering its radioactivity to kill the cancerous cells there. Iodine‑131 has an eight-day half-life, after which it decays into a stable isotope of the element xenon (xenon‑131). So, although the iodine‑131 is dangerous to healthy cells, the bulk of the exposure is limited to a few weeks, which is why it can be intentionally introduced into the body to treat the disease.
Iodine-129, however, has a half-life of nearly 16 million years. Iodine‑129 released into the atmosphere from a nuclear explosion can cause contamination in plants, soils, and rivers. Additionally, iodine‑129 in waste from nuclear processing can leach into the environment from leaky storage containers. Although one might expect it to become more dilute as it spreads through the environment, which would reduce the risk of exposure for humans, it could alternatively concentrate in growing grasses or plants and then enter the food web. For instance, milk from cows that eat grass in contaminated areas, or meat from fish that swim in contaminated water, can be carcinogenic to people.
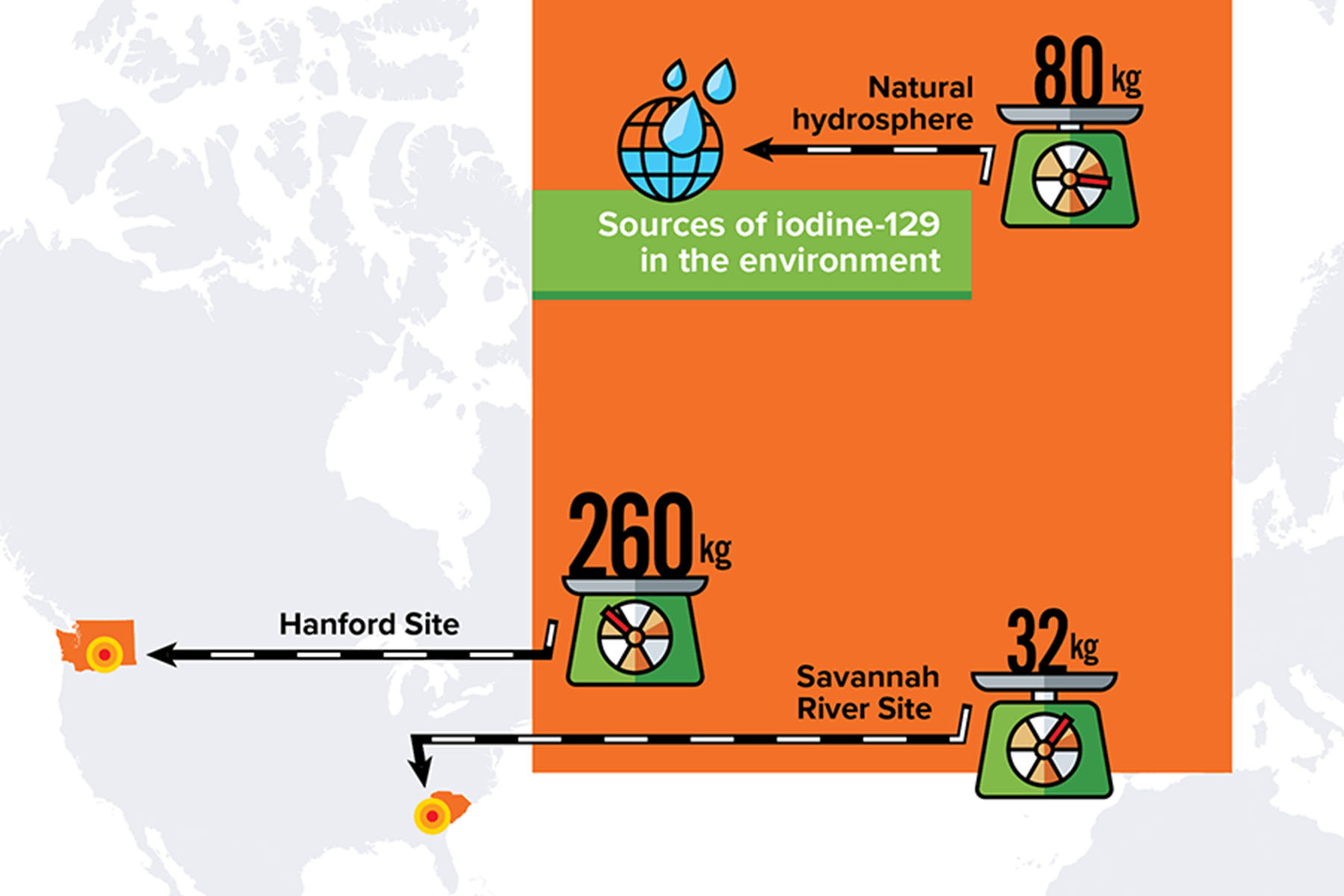
Moving target
During the Manhattan Project and the Cold War decades that followed, the United States produced large amounts of nuclear waste at weapons program sites such as Hanford in Washington state and Savannah River in South Carolina. For example, at the Hanford Site alone, there are currently about 260 kilograms of iodine-129 inventoried in the soil. (In contrast, the entire hydrosphere of the earth contains only about 80 kilograms of naturally occurring iodine-129). Iodine-129 is considered a significant threat at these legacy sites because of the possibility that it could spread through the environment and cause long-term damage to nearby populations. For this reason, the DOE has long funded studies to investigate the mobility of radioactive iodine and how to mitigate it.
The team Yeager works with, which is led by Dr. Peter Santschi from Texas A&M University at Galveston (TAMUG) and also includes Dr. Chen Xu from TAMUG, Dr. Daniel Kaplan from SRNL, and Dr. Patrick Hatcher from Old Dominion University, has been studying radioactive iodine mobility for more than ten years. The researchers began by looking at minerals found in the South Carolina soil that could bind to iodine-129 and perhaps affect its mobility, but early in their research the scientists realized there was a flaw in the models they were using. They had accounted for only one of several chemical states in which iodine can exist.
When radioactive iodine interacts with the environment, it can gain or lose electrons, which alters the way the iodine behaves. While an isotope such as iodine-131 or iodine-129 is defined by the number of neutrons present, the oxidation state is defined by adding or removing electrons. Each isotope of iodine or radioactive iodine can exist as seven different chemical “species,” depending on the oxidation state.
Nuclear power and weapons production both create iodine-129 primarily in the form of iodide, the state with one extra electron. Because of this, DOE models that have been used to predict the flow of iodine-129 through the environment are based on its mobility as an iodide compound, which can be easily carried by water through an ecosystem. However, depending on the surrounding environmental conditions, the iodine-129 does not always remain an iodide species.
For instance, if the environmental conditions are conducive to pulling off the extra electron, the iodide can join with a second iodide to make diatomic iodine, I2. Diatomic iodine is not particularly stable, so once this happens, many other reactions quickly follow. If oxygen is present, the I2 spontaneously becomes iodate, IO3-. Iodine can also bind to carbon found in natural organic matter to make various forms of organic iodine. When iodide changes in these ways, it can subsequently begin to stick to surrounding minerals, slowing its mobility through the environment. Yeager’s team began to realize that it needed to consider the speciation of radioactive iodine in the environment in order to accurately predict its movement, and ultimately, the corresponding risk.
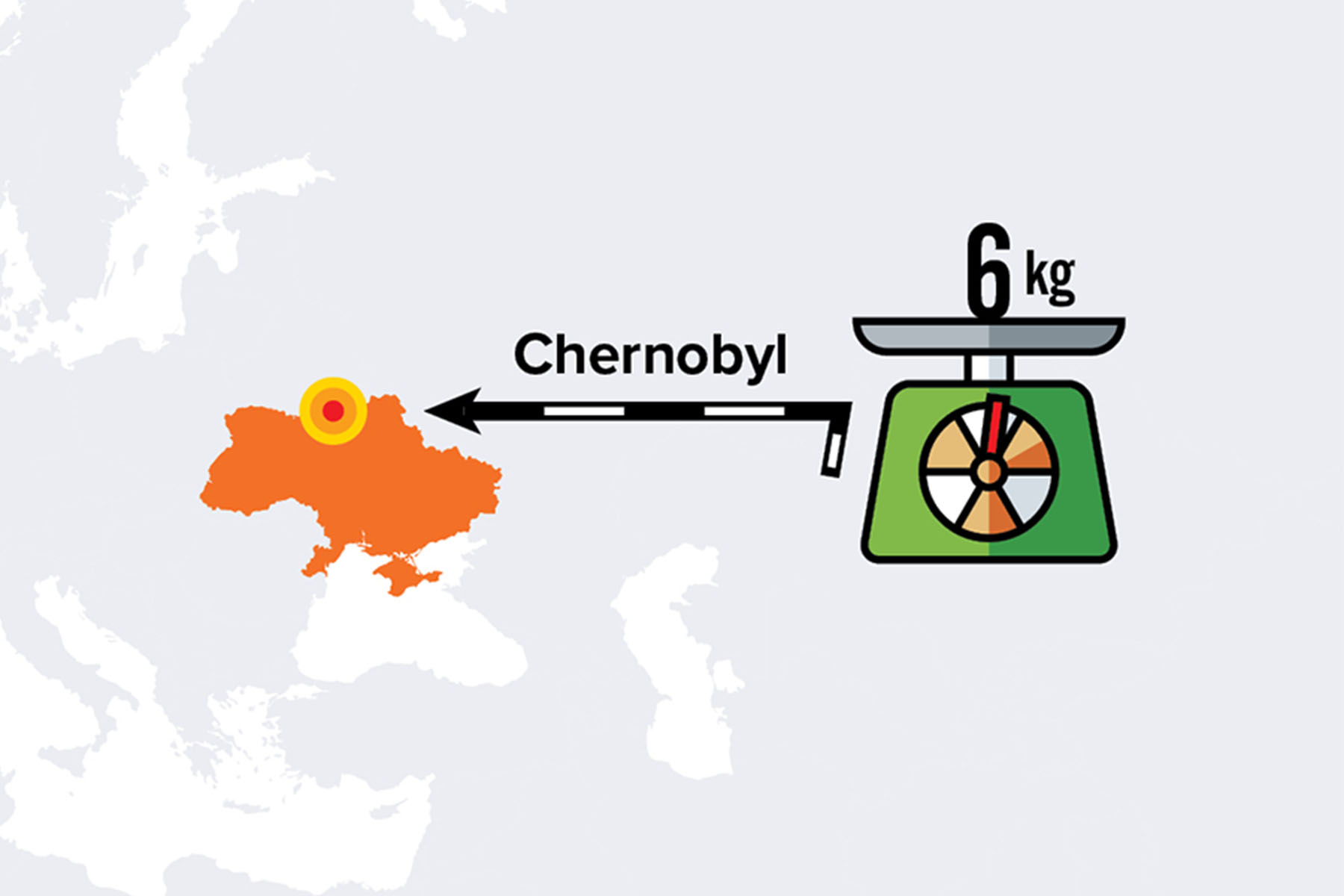
The significance of the speciation in contaminated soil is that in the form of iodide, the radioactive iodine is relatively mobile. Although more difficult to contain, radioactive iodide is readily diluted during natural transport through the environment, whereupon it does not pose a significant health risk. Conversely, iodate and organic iodine are much more likely to stick to the soil, and thus move much more slowly. This is both good and bad. If the radioactivity is contained in the soil in a known area and is moving very slowly, then it can be avoided, monitored, and effectively stored in place. The problem with this, however, is that dangerous levels of contamination are still present in the environment, awaiting the development of new, cost-effective clean up technologies.
Microbes in the melee
Although it had been previously thought that most subsurface waters at the Savannah River and Hanford sites did not have the right conditions for oxidizing iodide, the data began to show otherwise. Yeager and his colleagues spent years studying soil and water samples from Savannah River and Hanford and discovered that, in fact, the radionuclide species in the soil included iodate and organic iodine, not just iodide.
Radioactive iodine could spread through the environment and cause damage to nearby populations.
Santschi’s laboratory developed a novel approach that uses gas chromatography with mass spectrometry to determine iodine speciation in environmental samples. This was a technological breakthrough because it enabled his team to detect species at ambient concentrations, i.e, below 10 parts‑per‑million. (Earlier studies typically measured iodine at much higher concentrations, which were not environmentally relevant.) The team’s ability to detect various species of iodine led to a more thorough investigation of the contaminated areas. But it also led to more questions about what could be happening to the radioactive iodine in the soil. More specifically, it put the pressure on
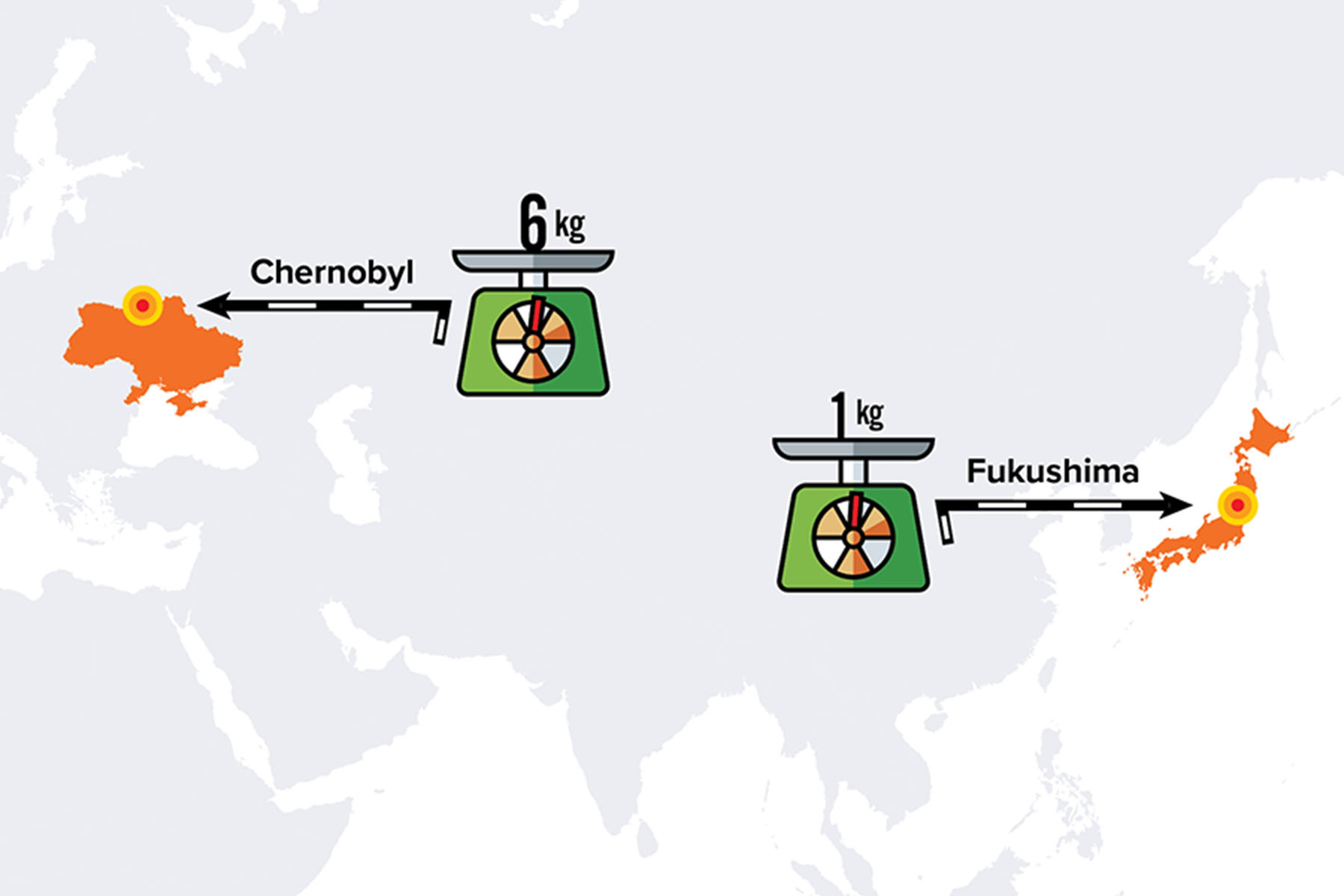
Yeager to find out if microbes might be involved with the changes in iodine species.
Yeager began a series of experiments, both at Los Alamos and with students at TAMUG, to culture microbes under varied conditions and observe whether or not they could oxidize iodide, and if so, what variables could impact the process? The experiments confirmed that microorganisms from varied environments could facilitate iodide oxidation in a number of ways. For example, some bacteria produce and secrete enzymes that are capable of directly catalyzing the oxidation of iodide. Additionally, the presence of microbes in the soil or groundwater can indirectly lead to iodide oxidation through the production and release of routine metabolic byproducts. For instance, Roseobacter AzwK-3b bacteria oxidize naturally occurring manganese into manganese oxides, which then act on iodide to make diatomic iodine—which is readily converted to iodate or organic iodine. Another indirect mechanism of iodide oxidation the team observed was when Roseobacter make reactive oxygen species (other chemically reactive molecules that contain oxygen), which similarly facilitate the conversion of radioactive iodide to iodate or organic iodine.
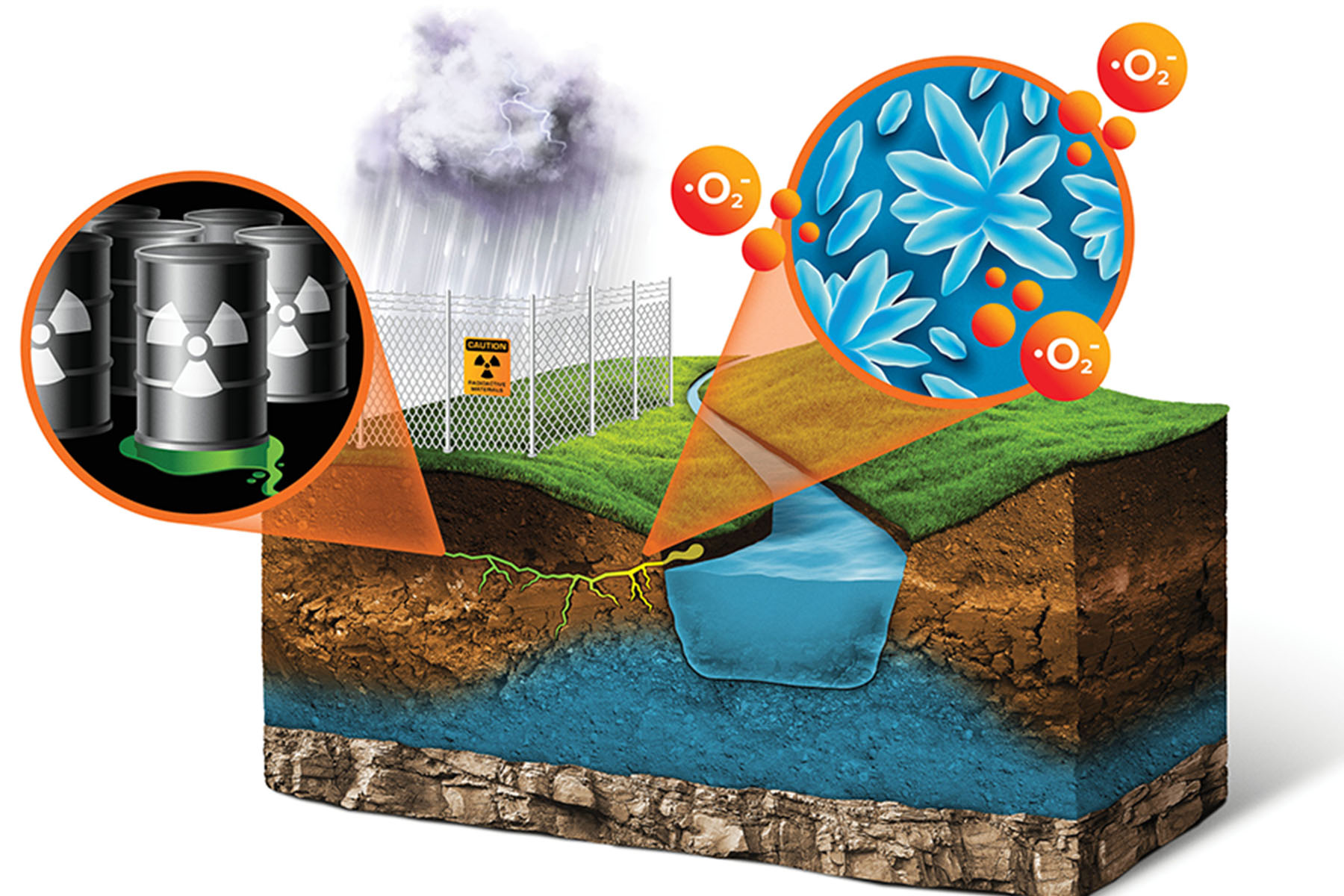
In 2011, the Fukushima Daiichi nuclear power plant in Japan experienced a meltdown after a major earthquake and subsequent tsunami. The meltdown resulted in a catastrophic release of radioactive material, including radioactive iodine. Yeager’s team has collaborated with two universities in Japan and one in South Korea to examine the terrestrial and aquatic environments surrounding Fukushima. Their data further confirm the Hanford and Savannah River finding that natural organic matter plays a key role in controlling the fate of radioactive iodine in the environment. The team also examined deposits of plutonium that had been released at Fukushima and got similar results regarding the important role of organic matter. Currently, the team is analyzing the relationship between plutonium and organic matter in sediments from a reservoir near Nagasaki, Japan, representing some of the oldest plutonium on Earth—contamination left over from the dawn of the nuclear age.
Living with legacy waste
Yeager and his colleagues have shown that each type of radioactive contamination reacts differently with natural organic matter in the environment. For instance, the team’s plutonium studies suggest that interactions between natural organic matter and plutonium can either immobilize or re-mobilize the radioisotope, depending on the conditions. This makes cleanup strategies even more complex, as each contaminant and its unique relationship with the environment needs to be more thoroughly researched than was previously realized.
If the radioactivity is contained in the soil in a known area, moving slowly, then it can be avoided, monitored, and stored in place.
Working with Los Alamos plutonium geochemist Hakim Boukhalfa and plutonium analytics expert Kate McIntosh, Yeager’s team hopes to gain a deeper understanding of the interaction between radionuclides, minerals, and natural organic matter. Using a confocal x-ray fluorescence microscopy technique pioneered at Los Alamos, the scientists have begun to generate 3D maps of the elements surrounding plutonium bound to soil particles from Savannah River. Such images could help the researchers co-locate plutonium with other elements, such as carbon and iron, to further reveal the chemistry that controls its movement in the environment.
Through these various studies, Yeager and his colleagues have begun to identify how inducing certain conditions could potentially serve as remediation techniques. With respect to radioactive iodine, the team’s discovery that oxidation can impact iodide mobility suggests that remediation treatments aimed at intentionally stimulating aerobic microbial activity in contaminated aquifers could be used to immobilize iodine‑129 in place.
“The oxidative processes of iodine are complex,” says Yeager, “but if oxygen is readily available and there is enough organic matter present, the microbes will facilitate the formation of relatively immobile iodine complexes.”
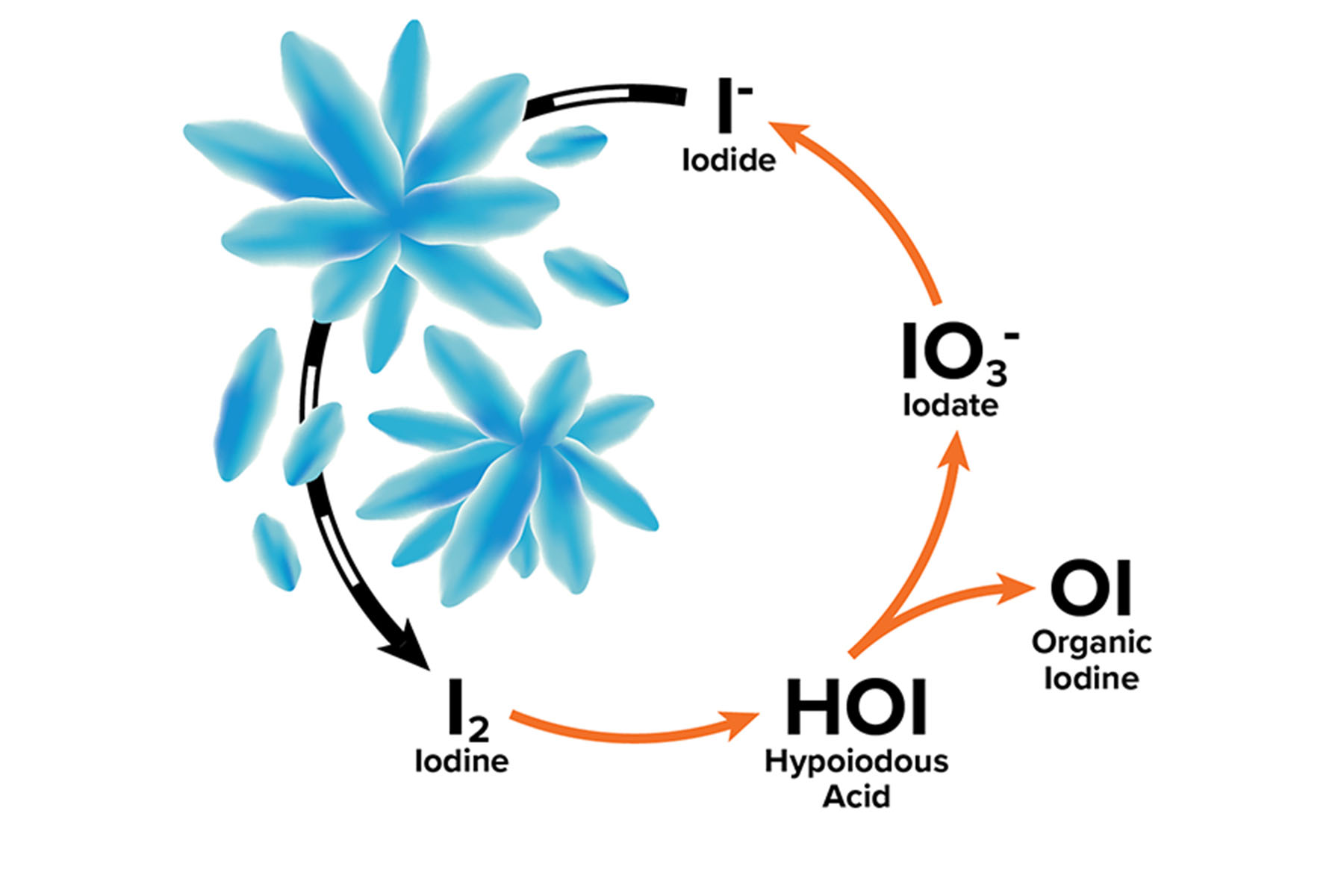
In addition to improving remediation, the evidence gathered on iodine-129 oxidation could improve modeling techniques used to predict the transport of radioactive iodine in various oxidative states, not just iodide. This would help scientists and governments plan for the future by more accurately predicting both the movement of the radionuclides through the environment and their potential impacts on human health.
Depending on the availability of microbes, other approaches to immobilizing iodide are also being considered. One uses silver to halt radioactive iodine by forming insoluble metal iodides. Another stimulates calcite precipitation in aquifers containing high levels of soluble carbonate, including the Hanford Site, thereby incorporating iodide into the rocky calcite minerals.
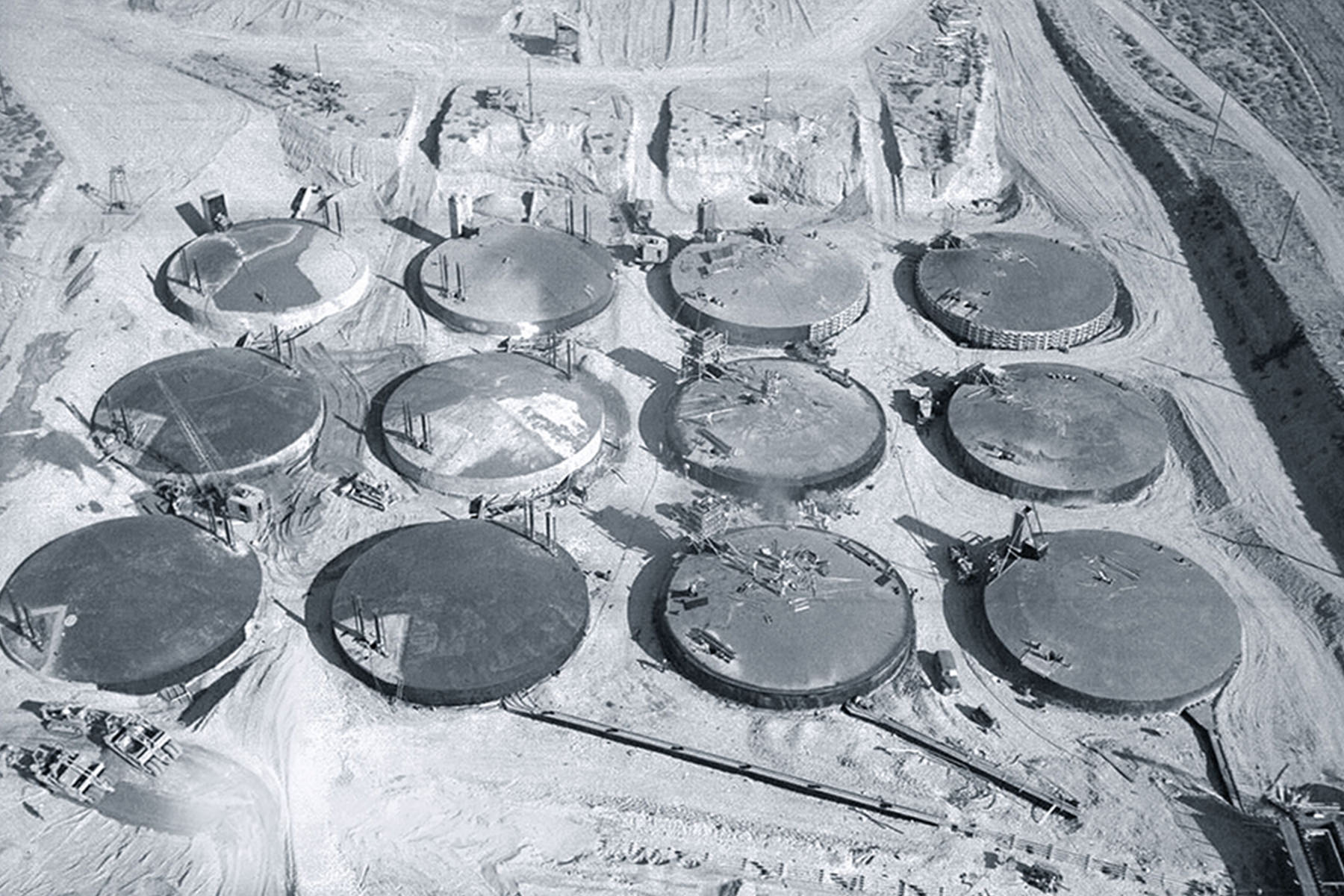
of radioactive waste that was stored in tanks like these. Recent discoveries of leakage of radioactive material has prompted a major environmental cleanup of the area and numerous studies to understand the risks of the radioactive contamination.
In this day and age of Snapchat messages and tweets, it often seems as though nothing lasts forever—yet nuclear waste is something that effectively does. A 16-million-year half-life for iodine-129 is a daunting reality for those whose job it is to clean up the nuclear legacy. The good news is that ultimately, everything on the planet gets recycled or changed into something else—it’s just a question of how long it takes and whether the new form or concentration is more or less harmful than the old one. For their part, Los Alamos scientists and collaborators are beginning to develop improved strategies by learning about our environment and studying the adaptations of Earth’s smaller and more numerous inhabitants. Suffice it to say, nature usually has an answer.